Research Article
Creative Commons, CC-BY
Protection of Episodic Memory by Neuroprotective Peptide Treatments in Sprague-Dawley Rats Subjected to Hypoxic Exposure
*Corresponding author: Victor Chan, Applied Biotechnology Branch, Airman Bioengineering Division, Airman Systems Directorate, 711 Human Performance Wing, Air Force Research Laboratory (711 HPW/RHBB), Wright Patterson AFB, OH 45433, USA.
Received: August 08, 2020; Published: September 09, 2020
DOI: 10.34297/AJBSR.2020.10.001496
Abstract
This study aimed to demonstrate successful protection of episodic memory function by intranasal instillation of neuroprotective peptides delivered prophylactically using a rodent model. Aerospace professionals frequently experience hypoxia, which may be preceded or followed by hyperoxic exposure. These conditions have both short- and long-term impacts on neurological and cognitive functions, resulting in impairments of spatial and working memory, concentration, and attention shifting. To test memory protective activities of ADNP-8 (also known as NAP/Davunetide) and Semax under hypoxic conditions, male Sprague-Dawley rats were treated with one of these peptides daily for four weeks. During the last five days of peptide treatment, rats were exposed daily to normobaric hypoxia (7.5% O2) or oscillatory hypoxia-hyperoxia (10 cycles of 95% ↔ 5% O2). Episodic memory performance was assessed using the novel object recognition (NOR) test immediately after the exposure. Rats exposed to hypoxia or oscillatory hypoxia-hyperoxia showed impaired performance in the NOR test, which could be prevented by ADNP-8 or Semax treatment. Consistent with the physiological effect of hypoxia, blood haemoglobin and erythropoietin levels were significantly altered in all exposed animal groups, independent of the exposure conditions or the peptide treatments they received. ADNP-8, but not Control Peptide, was able to abolish the change in VEGF and erythropoietin in hypoxia- and oscillatory-exposed rats, respectively. On the other hand, the changes in episodic memory seem to be unrelated to overt damage in the blood-brain barrier, since no significant change in the S100B level was detected in any experimental group with hypoxia/hyperoxia exposure. These results suggest that intranasal administration of neuroprotective peptides may be used as prophylaxes to prevent the impairment of episodic memory induced by hypoxia and oscillatory hypoxia-hyperoxia.
Keywords: ADNP-8, Semax, Hypoxia, Hyperoxia, Episodic memory, Novel Object Recognition, Hemoglobin, Erythropoietin, Vascular Endothelial Growth Factor
Introduction
Hypoxia is a condition of inadequate oxygen supply to the cells, tissues, or organs in the body. It may result from poor diffusion of oxygen across the pulmonary epithelium due to a low partial pressure of oxygen (ppO2) in the inspired air [1]. Oxygen availability decreases with the declines of barometric pressure (BP) as altitude increases. For example, at sea level, BP is 760 mm Hg (normobaric) and the ppO2 is 159 mm Hg, but at 3,048 m above sea level, BP is 523 mm Hg (hypobaric), and ppO2 is 110 mm Hg. Hypobaric hypoxia is a persistent threat for military personnel [2,3]. Pilots and aircrews utilize a variety of airborne vehicles including unpressurized helicopters operating below 3,048 m, fighters that fly above 7,620 m, with positive-pressure, supplemental oxygen, and surveillance aircrafts that can reach altitudes above 21,336 m, requiring fully pressurized suits similar to astronauts [2,3]. The lack of sufficient oxygen induces a cascade of molecular, cellular and physiological responses aimed to restore oxygen delivery and utilization in tissues [4]. Low oxygen availability impairs brain function; acute hypoxia degrades cognitive performance and motor tasks, while sustained hypoxia can cause lasting impairments of memory function, concentration, attention shifting, and motor control [5]. Cognitive impairment has been demonstrated at simulated altitudes from 2,438 m to 10,000 m [6, 7]. Negative impacts on pilots’ performance and cognitive functions have been observed, despite the subject’s affected reporting confidence in their abilities [8]. Furthermore, memory deficits persisted after returning to sea level [9]. Since deterioration of psychomotor performance starts at 2,438 m, commercial aircraft cabins are pressurized to simulate lower altitudes. On the other hand, military aircrafts are pressurized to a lesser extent and are more susceptible to a loss of cabin pressure, thus requiring oxygen supplementation to prevent the adverse effects of hypoxic events [10]. The percent of oxygen in the supplemental air is increased with the altitude up to 12,192 m, above which positive pressure breathing of 100% oxygen is necessary [11], but hypoxic mishaps still occur, most commonly resulting from faulty oxygen delivery at altitudes between 3,000 and 6,000 m [12,13]. In order to recover from a hypobaric hypoxia event, a pilot is expected to descend to a lower altitude (that has a higher BP) and/or increase the amount of oxygen in the inspired air, often to 100%. Breathing pure oxygen may resolve the symptoms of hypoxia, but it can also result in hyperoxia, a condition of excess oxygen in the tissues. Extended exposure to high oxygen levels can cause oxygen toxicity that damages the respiratory tract. The effects of hyperoxia on the nervous system increase with time, beginning with small muscle twitching, followed by vertigo, nausea, clumsiness, and then convulsions [14]. Both hypoxia and hyperoxia can induce cellular oxidative stress by increased levels of reactive oxygen species, resulting in cellular damage and altered tissue functions [15,16]. Brief exposure to 100% oxygen, however, reverses hypoxia and may have immediate, short-term, cognitive benefits including improved memory and reaction time [17, 18].
Given the prevalence of hypoxic events in the military setting [14], it is of interest to develop new methods or agents that can prevent the adverse effect of hypoxia on memory performance in order to improve the resilience of pilots and aircrews against this stressor. Herein we report on the potential of two neuroprotective peptides to prevent such negative effects on cognition. The 8-amino acid fragment of activity-dependent neuroprotective protein (ADNP-8, also known as NAP or Davunetide) can ameliorate oxidative stress and stabilize microtubules, thereby preserving neuronal viability and functions [19]. Semax is a synthetic analogue of residues 4-10 of the adrenocorticotropic hormone (ACTH); it likely exerts its neuroprotective activities by modulating the expression of neurotrophic and/or immune factors [20,21]. These peptides were administered prophylactically to Sprague-Dawley rats, by intranasal instillation, a method commonly used to target the central nervous system and successfully used for both Semax and ADNP-8 [22,23]. The peptide-treated rats were exposed daily to normobaric atmospheres with altered oxygen content: 60 minutes of hypoxia (ppO2 = 57 mm Hg) or 10 cycles of oscillating hypoxia-hyperoxia (ppO2 = 722 mm Hg ↔ ppO2 = 38 mm Hg), during the last five days of peptide treatment. Learning and memory were tested using the novel object recognition (NOR) test immediately following hypoxic/oscillatory exposure, on the last two days of the 5-day exposure period. NOR test is a common behavioural test used to assess the formation and retrieval of episodic memory of rodents, taking the advantage of their innate curiosity for exploring a novel stimulus. Physiological markers of hypoxic response and organ injury were examined, including hematocrit, haemoglobin, S100 calcium binding protein (S100B), erythropoietin (EPO), vascular endothelial growth factor (VEGF), angiotensin II; 1,3-bisphosphoglyceric acid (1,3-BPG) and 2,3-bisphosphoglyceric acid (2,3-BPG), for evaluating the correlations with the result of the NOR test. While rats subjected to hypoxia or oscillatory hypoxia-hyperoxia showed impaired NOR performance, ADNP-8 treatment effectively protected memory performance of rats subjected to hypoxic or oscillatory hypoxic-hyperoxic exposure. On the other hand, Semax treatment had a slightly different protective profile towards these exposure conditions. While it can protect against oscillatory hypoxia-hyperoxia, its effect against hypoxia is highly evident and reached a positive trend. The result of physiological markers analysis confirmed the effect of hypoxia/hyperoxia and suggests that some of these physiological responses may be modulated by peptide treatments. In summary, these results demonstrated that prophylactic treatment of ADNP-8 or Semax can successfully protect episodic memory function of Sprague Dawley rats.
Materials and Methods
Animals and Husbandry
The study protocol was approved by the Wright-Patterson Air Force Base Institute of Research, Institutional Animal Care and Use Committee (IACUC), and by the U.S. Air Force Surgeon General’s Office of Research Oversight and Compliance. The experiments reported herein were conducted in compliance with the Animal Welfare Act and in accordance with the principles set forth in the "Guide for the Care and Use of Laboratory Animals," Institute of Laboratory Animal Research, National Research Council, National Academies Press, 2011. They were conducted in a facility accredited by the Association for the Assessment and Accreditation of Laboratory Animal Care (AAALAC).
The animal subjects were Specific Pathogen Free (SPF), Male, Sprague Dawley Rats (Rattus Norvegicus), CRL: CD (SD) (Charles River Laboratories, Wilmington, MA). They were 6 weeks old on arrival and placed in quarantine for 2 weeks while being tested for ten specific rodent pathogens. They were examined visually at least twice per day during the study for signs of distress. Only males were used to avoid the compounding effect of estrous cycles and gender-specific behavioural differences and responses to hypoxia. Rats were socially housed (2/cage) in clear plastic cages with conventional bedding (CellZorb, Cincinnati Lab Supply, Cincinnati, OH) and provided tunnels, nesting material, and nylon bones for enrichment. Food (LabDiet Formulab Diet 5008, Cincinnati Lab Supply) and water were freely available. The animal rooms were climate-controlled with a 12-h light/dark cycle (lights on at 0600).
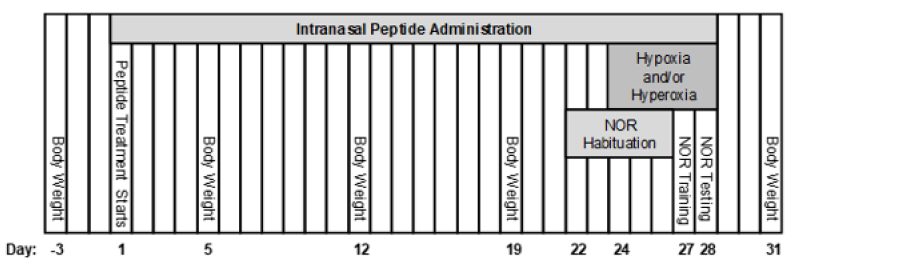
Figure 1: Experimental Design. Each vertical rectangle represents one day. Sprague-Dawley rats were treated daily with one of the peptides tested (Control, ANDP-8 or Semax) from Day 1 to Day 28. Hypoxic or oscillating hypoxic-hyperoxic exposure of animals were from Day 24 to Day 28. Memory performance, assessed using the novel object recognition (NOR) test, was conducted at Days 27 and 28, for training and testing, respectively. Collection of samples for biochemical analyses was performed at Day 31.
Experimental Design
The study was conducted in 2 separate experiments, according to the exposure conditions: hypoxia and oscillatory hypoxia-hyperoxia, and both experiments contained three (3) peptide treatment groups, Control Peptide, ADNP-8 and Semax (see Peptide Treatment section below for details). Eight rats were assigned to each group (using a computerized random number generator), and treated daily by intranasal administration of the respective peptide for 28 days. During the last five days of the peptide treatment period, rats were exposed daily to normobaric hypoxia or 10 cycles of normobaric oscillatory hypoxia-hyperoxia (see Hypoxic and/or Hyperoxic Exposures section below for details). Habitation of animals to the NOR test environment was performed at Days 22-26 (i.e. 1-5 days before NOR testing). On the last two days of exposure, NOR training and testing were performed. Naïve Control rats (with no exposure and no peptide treatment) were included in both experiments as reference for evaluation of the effects of exposure and peptide treatment. Thus, each experiment contained four animal groups: Naïve Control and three exposed groups treated with Control Peptide, ADNP-8 or Semax. All animals were euthanized at Day 31 (three days after the NOR test) for sample collection. Days with multiple tasks were handled in the following order: peptide treatment, hypoxic/oscillatory exposure, and habituation/NOR test. The experimental design is shown in Figure 1.
Peptide Treatment
Peptides used in this study were custom-synthesized, with a purity of >98%. ADNP-8 and Semax were supplied by Selleckchem (Houston, TX). The Control Peptide (supplied by Thermo Fisher Scientific, Carlsbad, CA) is an empirically designed 9-mer peptide, which only contains small amino acids glycine and alanine and is expected to have minimal biological activity. The information of these peptides is summarized in Table 1. The peptides were dissolved in phosphate buffered saline, pH 7.0 (PBS) (Thermo), at 1.25 mg/mL (ADNP-8) or 6.25 mg/mL (Semax and Control Peptide) and administered daily to the corresponding groups for 28 days by intranasal instillation. The dose volume for each rat (0.02 mL) was calculated weekly based on individual body weight (BW) and divided evenly between the two nares.
Hypoxic and/or Hyperoxic Exposures
Exposure of rats to normobaric hypoxia or oscillatory hypoxia-hyperoxia were accomplished in custom-built, clear, polycarbonate chambers approximately 40 cm wide, 35 cm long, and 21 cm high (Coy Laboratory Products, Grass Lake, MI), which provides real-time control over the oxygen content of the internal gas. A specific oxygen concentration was set for each exposure condition, with nitrogen used to complete the normobaric atmosphere, using medical grade gas cylinders (Airgas, Dayton, OH). Two rats (cage mates) were transferred to the chamber and exposed to the selected atmospheres. Hypoxia exposure was 7.5% oxygen (ppO2 = 57 mm Hg, corresponding to the oxygen level at an altitude of 7800 m) for 60 minutes. This was the lowest oxygen concentration that could be tolerated by rat subjects as determined in a serious of preliminary studies. The oscillatory hypoxic-hyperoxic exposure started with 8 minutes at 95% oxygen (ppO2 = 722 mm Hg), followed by 10 cycles of 5% (ppO2 = 38 mm Hg) and 95% oxygen for 3 minutes and 8 minutes, respectively. As above, this cycling profile was determined to be the most severe condition tolerable by rat subjects in preliminary tests. It normally takes less than 2 minutes to complete each transition of the oxygen concentration from high to low (and vice versa) in the chamber, and the total exposure lasted about 2.5 hours. Rats received total five daily exposures from Day 24 to Day 28 (Figure 1). During exposure, rat subjects were monitored closely for signs of distress.
Novel Object Recognition (NOR) Test
Rats were neither subjected to NOR testing following the procedure described by Barnes et al. [24] at Days 27 and 28 after the daily hypoxic or oscillatory hypoxic-hyperoxic exposure. The NOR field was an open-top, 60-cm long and wide, black plastic box with 40-cm walls. Activity was recorded by video camera and analysed using Ethovision XT 12 tracking software with a three-point body-tracking module (Noldus, Leesburg, VA). Fluorescent tubes supplied light between 3 and 6 lux to the NOR field. Habituation lasted 30 minutes daily in the testing room and ten minutes in an empty NOR field, starting at Day 22. Exploration time for training (Day 27) was ten minutes, and for testing (Day 28) was five minutes. Objects with similar sizes were used: rubber duck, round wooden tower, square plastic tower. Familiar and novel objects were assigned randomly while ensuring an equal number of instances for each object used as the novel object, as well as equal left and right placements of the novel object, in a counter-balanced manner. The objects were placed in equal distances from the opposite corners of the field and held in place by Velcro. The exploration zone included a 2-cm buffer zone around each object. Rats passed the test if they achieved a positive discrimination ratio (DR) as determined using the formula below [25]:

The magnitude of the DR along with the percent of time exploring each object served to indicate the degree of novel object preference (and how it is affected by hypoxic/hyperoxic exposure and peptide treatment). Age-matched, male Naïve Control rats with no exposure and no peptide treatment, housed in clear plastic cages and placed near the Coy chambers for 5 days, 2.5 hour/day (during the exposure of the experimental groups) were included in both experiments as reference.
Physiological Response to Hypoxic/Hyperoxic Exposure
Rats were euthanized by exsanguination or decapitation under Ketamine/Xylazine anesthesia, three days after the final exposure and NOR test. Whole blood was collected in K3EDTA Vacuette tubes (Greiner, Monroe, NC) for the measurements of hematocrit, hemoglobin, 1,3-BPG and 2,3-BPG. The portion for the hematocrit was processed using the StatSpin MP (Iris, Chatsworth, CA) and measured using the CritSpin Digital Hematocrit Reader (Iris). Red blood cells (RBCs) were collected by centrifugation and lysed using RBC lysis buffer (Abcam, Cambridge, MA) for the determination of 1,3-BPG and 2,3-BPG concentrations, using analyte-specific, rat ELISA kits (MyBiosource, San Diego, CA). Hemoglobin was measured using the Hemoglobin Assay Kit (MilliporeSigma, St. Louis, MO). Plasma was isolated by centrifugation of blood collected in K3EDTA Vacuette tubes and stored at -80 °C for the quantification of angiotensin II (Ang II) and vascular endothelial growth factor (VEGF), using specific ELISA kits (MyBiosource, San Diego, CA). Serum was isolated by centrifugation of post-mortem blood collected in BD Vacutainer SST tubes (BD, Franklin Lakes, NJ) and stored as above for the analysis of S100 calcium-binding protein B (S100B) and Erythropoietin (EPO), using ELISA kits from MyBiosource (San Diego, CA) and Biomatik (Wilmington, DE), respectively. Samples from Naïve Control rats were included in all assays.
Statistical Analysis
The data was analysed using Prism 7 for Windows, version 7.05 (GraphPad Software, La Jolla, CA). The means were compared using 2-way ANOVA with treatment and exposure as factors (alpha = 0.05). The Naïve Control rats of the two experiments were pooled for the analysis of physiological markers. When ANOVA revealed a significant effect, the appropriate means were compared using the Tukey’s multiple comparison test to identify significant differences (alpha = 0.05). For an exposure effect, comparisons were made between the Naïve Control and exposed groups. For a treatment effect, the group with ADNP-8 or Semax treatment was compared with the Control Peptide group within the same experiment. Pairwise comparisons were also made between the exposures with the same peptide treatment. The change in body weight over time during treatment was analyzed by 2 methods: the difference in body weight over time was tested using linear regression and the percent change in body weight during the treatment period was analyzed by 1-way ANOVA. Organ weights were normalized by the body weight at Day 31.
Results
Physiological Effects of Hypoxia and Oscillatory Hypoxia-Hyperoxia
All peptide treatment groups of the hypoxia experiment gained weight normally during the entire experimental period, including the hypoxic exposure period (Figure 2A). In fact, their body weights were comparable with that of the Naïve Control (labelled as None/Open Air) at necropsy. The Control Peptide group had the highest body weight gain, followed by ADNP-8 and then Semax/Naïve Control. The difference in the slope of body weight gain over time was statistically significant between the Control Peptide and Semax groups (p=0.03), but only showed a positive trend between Control Peptide and ADNP-8 groups (p=0.09). All animal groups seemed to gain more weights during Week 2 - Week 3 and Week 4 - Necropsy than other periods. The reason for this is not clear. In oscillatory hypoxia-hyperoxia experiment, all peptide treatment groups also gained weight during the peptide treatment period. Similar to the result of the hypoxia experiment, the rats treated with either ADNP-8 or Semax gained less weight than the Control Peptide group. At Week 3, the difference in the percent body weight gain was statistically significant between Control Peptide and ADNP-8 treatment groups (p=0.04), as well as between Control Peptide and Semax treatment groups (p=0.03). However, all peptide-treated groups, especially the Control Peptide group, had much lower weight gains during the exposure period (Figure 2B). As a result, the body weights of all experimental groups were lower than that of the Naïve Control at necropsy. As shown in Figure 2C, the oscillatory hypoxia-hyperoxia groups gained less weight than the hypoxia groups during the week of exposures. Statistical analysis (2-way ANOVA) revealed a significant effect by exposure (p<<0.01) but not by peptide treatment (p=0.48). For the Control Peptide groups, there was a significant difference between the hypoxia and oscillatory hypoxia-hyperoxia exposures (p<<0.01). However for the ADNP-8 and Semax treatment groups, no significant difference between these two experiments was detected. This result suggests that exposure to oscillatory hypoxia-hyperoxia has a detrimental effect on body weight gain, which was partially prevented by ADNP-8 or Semax treatment, although these peptides, by themselves, could reduce body weight gains. One rat treated with control peptide died during oscillatory hypoxic-hyperoxic exposure, but the cause was not readily apparent based on gross pathological assessment.
Despite higher body weight gains in the hypoxia-exposed animals, their brain weights were lower than their counterparts of the oscillatory exposure experiment. The Control Peptide groups showed the most dramatic difference between the two experiments (Table 2), which is statistically significant (p<0.01). Comparison with the brain weight of the open-air Naïve Control showed that there is a significant decrease in the brain weight of the hypoxia Control Peptide group (p<0.05), highlighting the detrimental effect of hypoxia on the brain. On the other hand, oscillatory hypoxic-hyperoxic exposure showed no significant effect on the brain weight, and all exposed groups had brain weights very similar to that of the Naïve Control. Normalization of brain weight by body weight further confirmed the detrimental effect of hypoxia on the Control Peptide group; its normalized brain weight was significantly lower than that of oscillatory-exposed Control Peptide group (p<0.05). This effect was completely prevented by Semax treatment, and the brain weights of the Semax groups showed no significant difference between these two experiments. This result suggests a beneficial effect of this neuroprotective peptide. On the other hand, ADNP-8 treatment was only partially effective in preventing a loss of brain weight after hypoxic exposure. The brain weights (with and without normalization) of ADNP-8 groups were significantly different between hypoxia and oscillatory hypoxia-hyperoxia experiments (p<0.05). Besides the brain, all other organs investigated (including the heart, left lobe of the lung, liver and spleen) were not significantly affected by peptide treatments or exposure conditions (Table 2). Although the right lobes of the lung showed statistically significant effect of exposures, they were no longer significant after the correction for multiple comparison.
Increased production of red blood cells, resulting in elevated hematocrit is a common response to hypoxia. However, the hypoxia- and oscillatory-exposed groups only showed very small, insignificant changes in hematocrit, compared with the open-air Naïve Control (Table 3). On the other hand, significant changes in haemoglobin were observed in most experimental groups. In the hypoxia experiment, Control Peptide group showed a significant increase in the haemoglobin level, compared with the Naïve Control. While Semax treatments did not prevent this effect, ADNP-8 treatment further increased the level of haemoglobin after hypoxic exposure.
Interestingly, all three peptide treatment groups are significantly different from their counterparts of the oscillatory exposure experiment (all with p<0.001). In the oscillatory-exposed animal groups, Control Peptide treatment resulted in a significant decrease in the level of haemoglobin, compared with the Naïve Control. Interestingly, this effect was exacerbated by ADNP-8 and Semax treatments, resulting in a larger decrease in hemoglobin. Detailed analysis revealed that the changes in haemoglobin were much larger than that in hematocrit in all experimental groups. On average, hypoxia-exposed animals had an increase of <10% in hematocrit, while they had an increase of ~50% in hemoglobin. Similarly, oscillatory-exposed animals had decreases of ~6% and ~30% in hematocrit and hemoglobin, respectively. As a result, the hematocrit to hemoglobin ratio decreased by ~30% and increased by ~30% in the hypoxia- and oscillatory-exposed animals, respectively. To further evaluate the implication of these results, the ratio of hematocrit (vol. % of RBC) to hemoglobin (in g/dL) was determined. As shown in Table 3, hypoxia and oscillatory hypoxia-hyperoxia showed opposite effects on the ratio of hematocrit to hemoglobin, with the former causing an increase while the latter inducing a decrease in this ratio (Table 3). Although the mechanism(s) for this result is not completely clear, changes in the mean corpuscular volume and/or mean corpuscular hemoglobin (concentration) might be involved. However, this was not further investigated in this study.

Table 1: Peptide Dosage and Number of Rats in Each Experimental Group. The amino acid sequence (single-letter abbreviations), purity (provided by suppliers) and intranasal dose concentration of each peptide used for treatment are shown. Male Sprague-Dawley rats were treated with the peptides indicated in the first column and exposed to the oxygen concentration specified for each experiment.
*One animal died during exposure.

Table 2: Normalized Organ Weights of Naïve Control and Experimental Groups. The weights of organs investigated were determined at the end of the experiment and normalized by body weight. Values shown are mean ± standard deviation. The number of animals were the same as in Table 1. Values with the same superscripted alphabet are statistically significantly different (2-way ANOVA, Tukey’s multiple comparison test, alpha = 0.05).
*Significant exposure effect by ANOVA, but not significant after multiple comparison correction.
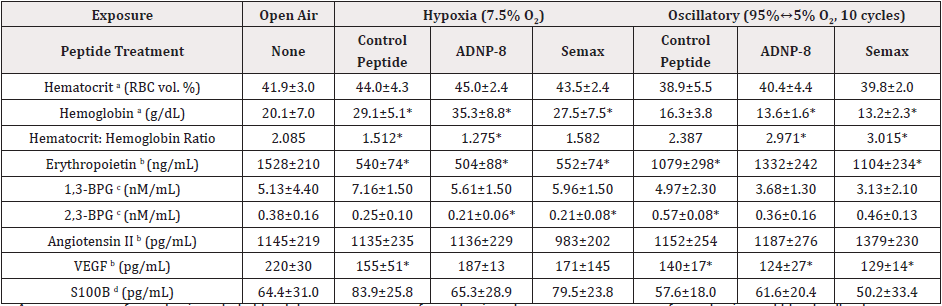
Table 3: Markers of Physiological Responses to Hypoxic and Oscillatory Hypoxic-Hyperoxic Exposures. The analytes investigated were determined using the blood samples collected at the end of the experiment. Values shown are mean ± standard deviation. The number of rats in each group was the same as in Table 1. An asterisk (*) indicates the value is significantly different from the Naïve Control (2-way ANOVA, Tukey’s multiple comparison test, alpha = 0.05).
a: Assays were performed using whole blood; b: assays were performed using plasma; c: assay was performed using red blood cells; d: assay was performed using serum.

Table 4: Exploration Time and Distance Traveled by Naïve Control and Experimental Group in the Novel Object Recognition (NOR) Test. The average exploration time and distance traveled of animals during the NOR test were determined. Values shown are mean ± standard deviation. The number of rats in each group was the same as in Table 1.
a: Assays were performed using whole blood; b: assays were performed using plasma; c: assay was performed using red blood cells; d: assay was performed using serum.
EPO, a glycoprotein cytokine secreted by the kidney to stimulate erythropoiesis in the bone marrow, is commonly upregulated in response to hypoxia. As hypoxia and oscillatory hypoxia-hyperoxia exposures appear to have opposite effects on the level of hemoglobin, it is of interest to investigate how the level of EPO responded to these exposure conditions. As shown in Table 3, hypoxia-exposed groups showed decreased levels of EPO, which is significantly different from that of the Naïve Control. On the other hand, the EPO levels of oscillatory-exposed groups were only slightly lower than that of the Naïve Control. In addition, the EPO levels were significantly different between the two experiments for the Control Peptide and Semax treatment groups (both with p<0.05).
The binding of 2, 3-BPG to hemoglobin promotes the release of oxygen into surrounding tissues. Increased 1,3-BPG synthesis and its subsequent conversion to 2,3-BPG represents a normal physiological response to hypoxic exposure. Compared with Naïve Control, Control Peptide group showed a small increase in the 1, 3-BPG after hypoxic exposure, and this change was abolished by both ADNP-8 and Semax treatments. In the oscillatory hypoxia-hyperoxia experiment, rats treated with ADNP-8 and Semax, but not Control Peptide showed decreased levels of 1,3-BPG. However, it should be noted that none of these changes reached statistical significance threshold. All hypoxia-exposed groups showed a decrease in 2,3-BPG; only the ADNP-8 and Semax groups (but not Control Peptide group) were significantly different from the Naïve Control (p<0.05). In contrast, the oscillatory-exposed rats with Control Peptide treatment showed a significant increase in 2, 3-BPG, compared with the Naïve Control (p<0.05). This effect was reduced by ADNP-8 treatment, but not by Semax treatment, with the difference between the Control Peptide and ADNP-8 groups reached statistical significance threshold (Table 3). However, no significant change in bisphosphoglycerate mutase, an enzyme responsible for the catalytic conversion of 1, 3-BPG to 2,3-BPG, was observed in any experimental group, compared with the Naïve Control (data not shown).
Circulating angiotensin II is essential for arterial pressure elevation through its stimulation of vasopressin and aldosterone production and secretion, as well as its effect on vasoconstriction, following hypoxia exposure. As shown in Table 3, the concentration of Angiotensin II in the plasma was not significantly altered in any experimental group regardless of exposure condition and peptide treatment (Table 3). Consistent with this result, the serum level of copeptin, a surrogate marker for vasopressin [26,27] also showed no significant change in any experimental group, compared with the Naïve Control (data not shown).
Stimulation of the renin–angiotensin system by hypoxia promotes the expression of VEGF, which in turn stimulates angiogenesis and increases vascular permeability to enhance oxygen supply to tissues. Statistical analysis showed that the effect of peptide treatment on VEGF was not significant (p=0.83), but that of exposure conditions was (p<<0.01). VEGF was significantly decreased in all experimental groups in both experiments (p<<0.01), except the ADNP-8 and Semax groups with hypoxic exposure. Similar observations have been reported previously by other investigators [28,29]. It is likely that VEGF expression is mostly localized to the tissues affected, while its level in plasma/serum is down regulated [29]. Interestingly, the significantly decreased level of VEGF as observed in the Control Peptide group of the hypoxia experiment was reversed by the ADNP-8 and Semax treatments. In contrast to the hypoxia experiment, ADNP-8 and Semax treatments seemed to exacerbate the VEGF-depressing effect of oscillatory hypoxia-hyperoxia exposure that was detected in the Control Peptide group. The difference between hypoxia-exposed and oscillatory-exposed groups was statistically significant for both ADNP-8 and Semax (p<<0.01).
Damage to the blood-brain barrier is a common phenomenon of brain injury induced by hypoxic exposure. The serum level of S100B, a peripheral biomarker of blood-brain barrier permeability and CNS injury was determined. Compared with the Naïve Control, there was no statistically significant change in level of S100B between any experimental group, regardless of exposure conditions and peptide treatments, suggesting that the exposure conditions used in this study were not strong enough to cause overt blood-brain barrier damage (Table 3).
Effect on Novel Object Recognition Test Performance
Episodic memory function of Naïve Control and experimental groups was assessed using NOR test, which determines the ability of a rat subject to distinguish a novel object that has not been previously seen, from a previously encountered, familiar one. Rats with normal memory functions will naturally spend more time exploring a novel object than a familiar one, and the difference in exploratory time served as an indicator of episodic memory formation during training and retrieval during testing. Initially, the effect of exposure conditions and peptide treatments on the overall activity was assessed, and the result showed that the total object exploration time was similar among all animal groups (Table 4). The hypoxia-exposed, Semax-treated group had the longest average exploration time (72.9 sec.). The total distance traveled during the NOR test was also similar among all animal groups (Table 4), with the ADNP-8 and Semax groups of the hypoxia experiment traveling the longest distance. However, there is no significant difference in these parameters between any animal groups.
In the hypoxia experiment, the Naive Control group (labeled as Open-Air Control) spent approximately 60% and 40% of exploratory time with the novel and familiar objects, respectively (Figure 3A), and the difference in object exploration is statistically significant (p<0.05), resulting in an average DI of >0.2 (Figure 3B). Seven (7) rats of this group (n=8. i.e. 87.5%) passed the test while only one (1) failed (Figure 3C). Rats that were treated with Control Peptide explored the novel and familiar objects for about the same amount of time (Figure 3A). The average DI of this group was below zero (Figure 3B) and only three (3) rats (37.5%) passed (one of them only barely passed) the test (Figure 3C). In contrast, rats received ANDP-8 treatment had novel object exploration very similar to the Naive Control (Figure 3A) that they also showed statistically significant novel object preference (p<0.05). A similar conclusion can be drawn from the passing rate of the NOR test that more than 75% of the rats in this group passed the test (Figure 3C). As a group, they in fact had an average DI that is even slightly higher than that of the Naive Control (Figure 3B). Similarly, Semax treatment was also able to protect NOR function in rats that they also showed a positive trend (p=0.053) in novel object preference (Figure 3A). The average DI of Semax group is slightly lower than the Naive Control (Figure 3B), and only five (5) animals (62.5%) passed the NOR test (Figure 3C).
The result of the oscillatory hypoxia-hyperoxia experiment is very similar to that of the hypoxia experiment. The Naive Control group spent approximately 70% and 30% of exploratory time with the novel and familiar objects, respectively (Figure 3D), and the difference in object exploration is statistically significant (p<0.05). The average DI of this group is ~0.4 (Figure 3E), with all eight (8) animals passing the test (Figure 3F). Rats treated with control peptide actually spent more time with the familiar object than with the novel one (Figure 3D), consequently resulting in an average DI that was below zero (Figure 3E). Although four animals of this group passed the test (Figure 3F), two of them had a DI that is barely higher than zero (Figure 3E). As above, rats treated with ADNP-8 or Semax performed as well as the Naïve Control, spending ~70% of exploratory time with the novel object. While one rat of the ADNP-8 group failed the test (i.e. DI<0), all members of the Semax group passed the test (Figure 3E and 3F).

Figure 2: Body Weight Changes during Peptide Treatment and Hypoxic / Oscillatory Hypoxic-Hyperoxic Exposures. Animals gained weight during (A) hypoxia and (B) oscillatory hypoxia-hyperoxia exposure experiments, regardless of the nature of peptide treatments. The number of animals is the same as in Table 1. Animals of all experimental groups were weighed weekly, except that the Naïve Control group (labelled as “None/ Open Air”) was only weighed at necropsy. (C) The percentage of body weight gain during the week of exposure of all peptide treatment groups in both experiments. The asterisks (*) indicates the Control Peptide groups showing statistically significant difference between the two experiments.
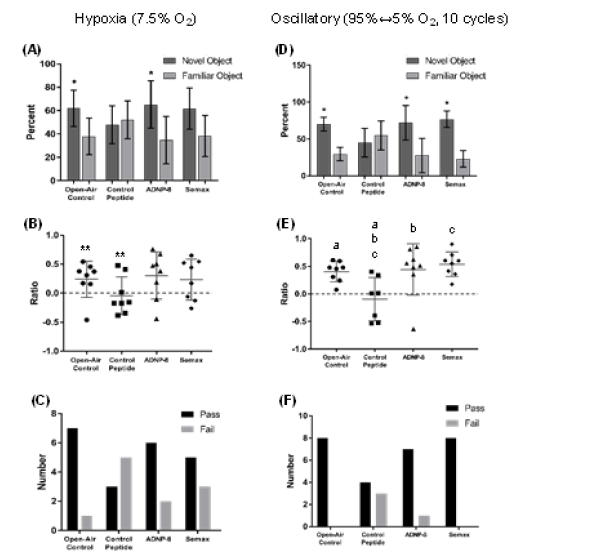
Figure 3: Results of Novel Object Recognition (NOR) Test. (A) and (D) The percentage of exploratory time spent with novel and familiar objects. (B) and (E) The discrimination ratio (DI) of each rat, along with the mean and standard deviation for each group. (C) and (F) The number of animals passing the test in the hypoxia experiment (A-C) and oscillatory hypoxia-hyperoxia experiment (D-F). The number of animals is the same as in Table 1. The asterisks (*) in (A) and (D) indicate the experimental groups whose exploration of novel object was significantly greater than that of familiar object (p<0.05). The double asterisk (**) in (B) indicates there is a positive trend in the difference between the Naïve Control (labeled as “Open-Air Control”) and Control Peptide groups (p<0.1). The difference between experimental groups labeled with the same alphabets in (E) are statistically significant (p<0.05).
It should be noted that the differences in exploration time, DI and number of animals passing the NOR test between Control Peptide and ANDP-8 treatment groups, as well as between Control Peptide and Semax treatment groups, are readily apparent in both experiments, especially in Figure 3E, in which the difference in DI between them reached statistical significance threshold. Taken together, these results demonstrated that both hypoxic and oscillatory hypoxia-hyperoxic exposures have detrimental effect on episodic memory in Sprague-Dawley rats. Furthermore, ADNP-8 or Semax treatments can effectively alleviate the negative impacts of static hypoxia and oscillatory hypoxia-hyperoxia exposures and protect episodic memory functions under these conditions.
Discussion
Due to the loss of cabin pressure and/or malfunctions of the oxygen delivery system, military pilots and aircrews may expose to abnormal concentrations of oxygen in the inspired air, resulting in physiological stresses associated with hypoxia and hypoxia-reoxygenation stresses. Hypoxic exposure can cause acute cellular damage and injury, which is followed by delayed neuronal death through a variety of mechanisms, including excitotoxicity, oxidative stress and apoptosis via different signal transduction pathways, resulting in neurological and cognitive impairments. Therefore, strategies that can protect these essential functions of Air Force personnel will ensure safe operation and return for timely therapeutic intervention to prevent long-term health problems. To achieve this goal, this study was designed to investigate the feasibility of protecting memory functions after exposure to altered concentrations of oxygen in the inspired air by prophylactic treatment with neuroprotective peptides, ANDP-8 and Semax [30-37], using an animal model. Studies of spatial, visual, and working memory deficits following hypoxic exposures have been demonstrated using the Morris water maze. Exposure conditions include acute and continuous [38], chronic continuous [39-43], acute intermittent [44], and oscillating, chronic intermittent [45, 46]. Hypoxia-induced memory dysfunction has also been demonstrated using a radial-arm maze [47], and an object displacement/replacement test [48] that is similar to the NOR test used in this study. Likewise, the result described here showed that novel object preference, an indicator of episodic memory function, was impaired in rats exposed to normobaric hypoxia and oscillatory hypoxia-hyperoxia, compared with the Naïve Control rats. More importantly, our results also showed that this performance deficit was effectively prevented by a prophylactic treatment of ANDP-8, but not the Control Peptide that has a random sequence of small amino acids such as glycine and alanine. Others have reported that ADNP-8 can protect the brain from a variety of insults; it prevented tissue damage, reduced mortality, prevented cognitive impairment, and improved neurobehavioral recovery in rodent models of traumatic brain injury [49], hypoxic-ischemic injury [50,51], and Alzheimer’s disease [52]. ADNP-8 also improved short-term memory in rats after 3 to 8 months of intranasal administration [53]. Like ANDP-8 treatment, Semax treatment also protected episodic memory function in rats exposed to oscillatory hypoxia-hyperoxia. Its protective effect was highly evident for hypoxia, although it only showed a positive trend (p=0.053). Other researchers have also reported on the neuroprotective effects of Semax that it reduced tissue damage and deficits in learning and memory caused by focal ischemic lesions in rats [33,54], and prevented Behavioral deficits caused by gestational exposure to valproic acid [55]. Combined with an opiate-like peptide, Semax prevented some of the growth and behavioral effects of gestational hypoxia [56]. Semax also improved learning and memory in rats exposed to heavy metals but caused deficits when used in the absence of the toxins or stressors [57,58]. In humans, Semax improved memory, attention, and brain circulation without negative side effects [reviewed in 22]. Consistent with their memory-protective functions, ANDP-8 and Semax were able to prevent the decrease in brain weight observed in the hypoxia-exposed rats treated with Control Peptide. As previous studies of ANDP-8 and Semax mainly focused on their activities in perinatal/neonatal hypoxia-ischemia [30-37], the results presented here thus extend the potentials of these peptides as preventatives or countermeasures against the adverse effects of hypoxia and oscillatory hypoxia-hyperoxia, major stressors in the aerospace environment. To realize the benefits of these agents, careful dose optimization will be needed, especially for Semax, due to its relatively narrow therapeutic window [30]. These efforts could ultimately result in successful development of neuroprotective prophylaxes containing one or both of these agents for Air Force pilots and aircrews.
Alleviation of hypoxia-induced memory deficits has been an area of active research [59,60]. A variety of agents, including organic compounds [38,44,61], neurohormones [62,63], plant extracts [41-43,64], cellular proteins [65-70], growth factors [71-74], peptides [30-37,75] and small interfering RNA [45,46] have shown different levels of neuroprotective activities under hypoxic conditions. The beneficial effects of pre- and post-exposure conditioning, voluntary exercise and environmental enrichment have also been demonstrated [48,76-80]. Among these interventions, EPO (and its analogues) are probably the most widely investigated agent. EPO exerts its anti-hypoxic activity through both hematopoietic pathways, as well as non-hematopoietic pathways that may involve neuroprotection and stimulation of recovery after injury [81-83]. However, the use of EPO is associated with certain risks, including myocardial infarction, stroke, venous thromboembolism, and even death [84]. The broad safety profiles of ADNP-8 and Semax represents a significant advantage over EPO and other erythropoiesis-stimulating agents.
Our results also showed that hypoxia and oscillatory hypoxia-hyperoxia induced significant changes in several physiological markers, including hemoglobin, EPO, 2, 3-BPG and VEGF. Some of these exposure-related changes were reversed by the ADNP-8 treatment. For instance, EPO was significantly decreased in rats with oscillatory hypoxic-hyperoxic exposure and Control Peptide treatment; ADNP-8 treatment partially reversed the decrease in EPO. ADNP-8 treatment also prevented the decrease in the VEGF that was observed in hypoxia-exposed rats with Control Peptide treatment. As EPO possesses both erythropoietic and neuroprotective activities [85,86], and VEGF can stimulate endothelial cell growth and migration, increase vascular permeability and induce angiogenesis [87], these results suggests that modulating the levels of these factors may have a role in the ADNP-8 mediated episodic memory protection.
Changes in the concentration of hemoglobin responding to abnormal levels of oxygen in the inspired air have been widely reported. It is up- and down-regulated under hypoxic and hyperoxic conditions, respectively, to regulate oxygen delivery to the tissues [88, 89]. Consistent with the reports in the literature, rats exposed to hypoxia showed increased levels of hemoglobin (Table 3). However, unlike the changes in EPO and VEGF, neither ADNP-8 nor Semax treatment was able to reverse the hypoxia-induced increase in hemoglobin that was observed in the Control Peptide group. Notably, no significant change in the hematocrit was detected in any experimental groups that showed alterations in the levels of hemoglobin. This effect was highlighted by the ratio of hematocrit to hemoglobin that hypoxia and oscillatory hypoxia-hyperoxia exposures showing opposite effects on this ratio, with the former causing an increase while the latter inducing a decrease in this ratio. As suggested by Siebenmann, et al., [89], the initial response to hypoxic exposure is an increase in circulating atrial natriuretic peptide and subsequently diuretic fluid loss and rapid contraction of plasma volume, resulting in increases in hematocrit and hemoglobin concentration. However, changes in the plasma volume alone should cause little change in the ratio of hematocrit to hemoglobin. Although the mechanism for the decrease in this ratio as observed in the hypoxia-exposed groups is not completely clear, it may be due to a decrease in the mean corpuscular volume with a concomitant increase in the total numbers of erythrocytes and an increase in mean corpuscular hemoglobin after hypoxia exposure. The high hemoglobin level in the ADNP-8 group suggests that this peptide is more effective than Control Peptide and Semax in stimulation the production of hemoglobin under hypoxia. On the other hand, the observation that decreased production of hemoglobin under oscillatory hypoxia-hyperoxia exposure was further exacerbated by ADNP-8 and Semax treatments suggests that these peptides may exert their memory-protective activities through the reduction of oxygen usage (and/or oxygen-induced injury). In contrast to the increased hemoglobin levels, the hypoxia-exposed groups had the lowest levels of EPO, compared with the oscillatory-exposed groups and the Naïve Control. This result might be due to delayed sample collection occurred three days after the final exposure, and the EPO level might have been downregulated by the feedback control mechanism [90] during the recovery period.
Other physiological markers investigated in this study (such as the ratio of 1,3-BPG : 2,3-BPG that influences the binding affinity of oxygen to hemoglobin [91], angiotensin II – an important player that regulates the fluid/electrolyte balance and blood pressure [92], and S100B – an peripheral marker of blood-brain barrier damage [93,94]) did not show any significant changes. This may be due to the normobaric exposure condition used in this study or delayed sample collection; these represent major limitations of the current study. In order to study not only the effects of static hypoxia, but also that of oscillatory hypoxia-hyperoxia that requires the maintenance of a constant pressure during the transition between high and low oxygen levels, we decided to use a normobaric condition for both experiments throughout this study. The intention is to ensure that the results of the two experiments can be compared, without the influence of the confounding effect caused by air pressure fluctuations. We are aware that there has been some debate over the use of normobaric hypoxia to simulate high-altitude, hypobaric hypoxia, and that the temporal, physiological response to hypoxia at different barometric pressures appears to differ slightly. For example, a forty-minute exposure to 12% O2 or its equivalent, ppO2 = 91 mm Hg, resulted in greater hypoxemia, hypocapnia, and blood alkalosis under hypobaric conditions, but greater arterial oxygen saturation under normobaria [95]. Yet, after 24 hours at 13.4% O2 (ppO2 = 102 mm Hg), there was little difference between hypobaria and normobaria [96]. It seems that the pattern of change in blood parameters is different under the two exposure conditions, but over time, both exposures will cause very similar physiological changes and hypoxic symptoms [97]. Moreover, even though the physiological response is slightly different, both exposures inhibit working memory in the same manner [98]. The repeated, acute exposures to normobaric hypoxia and oscillatory hypoxia-hyperoxia used in this study should, therefore, be acceptable as surrogates for studying the cognitive effects of hypoxia/hyperoxia encountered by in aerospace professionals. In fact, this study found that oscillatory hypoxia-hyperoxia had the greatest impact on body weight, VEGF levels, and memory performance. This agrees with Malle, et al., [98] who reported that breathing 100% oxygen while recovering from hypoxic exposure hastened the return to normal vascular oxygen levels but caused slowing of the EEG and poor performance on cognitive assessments.
Conclusion
This study showed that both neuroprotective peptides tested in this study, ADNP-8 and Semax, have the potential to be used as prophylaxes against the adverse effects of abnormal oxygen concentrations in the inspired air. It is tempting to speculate that a combination of these peptides might result in a more robust protection or even an enhancement of cognitive functions. In aerospace careers, hypoxic/hyperoxic exposures can occur frequently and unexpectedly. Prophylactic agents and countermeasures that can protect aerospace professionals are essential for mission success. The results presented here highlight the value of establishing the safety and effectiveness of these neuroprotective peptides for future Air Force applications.
Acknowledgments
The authors wish to thank Dominique Brown, Kerrine LeGuin, and the AFRL Research Support Center staff for their technical assistance that made this study possible. This research was supported in part by an appointment to the Postgraduate Research Participation Program at the U.S. Air Force Research Laboratory, 711th Human Performance Wing, Airman Systems Directorate, administered by the Oak Ridge Institute for Science and Education (ORISE) through an interagency agreement between the U.S. Department of Energy and US AFRL. This report was cleared for public release on July 16, 2019 (Case Number 88ABW-2019-3237).
Funding
This research did not receive any specific grant from funding agencies in the public, commercial, or not-for-profit sectors.
References
- Carreau A, El Hafny Rahbi B, Matejuk A, Grillon C, Kieda C, et al. (2011) Why is the partial oxygen pressure of human tissues a crucial parameter? Small molecules and hypoxia. J Cell Mol Med 15(6): 1239-1253.
- Neuhaus C, Hinkelbein J (2014) Cognitive responses to hypobaric hypoxia: implications for aviation training. Psychol Res Behav Manag 7: 297-302.
- Jersey SL, Hundemer GL, Stuart RP, West KN, Michaelson RS, et al. (2011) Neurological altitude decompression sickness among U-2 pilots: 2002-2009. Aviat Space Environ Med 82(7): 673-682.
- Prabhakar NR, Semenza GL (2015) Oxygen Sensing and Homeostasis. Physiology (Bethesda) 30(5): 340-348.
- Hornbein TF (2001) the high-altitude brain. J Exp Biol 204(Pt 18): 3129-3132.
- Petrassi FA, Hodkinson PD, Walters PL, Gaydos SJ (2012) Hypoxic hypoxia at moderate altitudes: review of the state of the science. Aviat Space Environ Med 83(10): 975-984.
- Malle C, Quinette P, Laisney M, Bourrilhon C, Boissin J, et al. (2013) Working memory impairment in pilots exposed to acute hypobaric hypoxia. Aviat Space Environ Med 84(8): 773-779.
- Asmaro D1, Mayall J, Ferguson S (2013) Cognition at altitude: impairment in executive and memory processes under hypoxic conditions. Aviat Space Environ Med 84(11): 1159-1165.
- Nation DA, Bondi MW, Gayles E, Delis DC (2017) Mechanisms of Memory Dysfunction during High Altitude Hypoxia Training in Military Aircrew. J Int Neuropsychol Soc 23(1): 1-10.
- Harding RM, Mills FJ (1983) Aviation medicine. Problems of altitude I: hypoxia and hyperventilation. Br Med J (Clin Res Ed) 286(6375): 1408-1410.
- Pilmanis AA, Sears WJ (2003) Physiological hazards of flight at high altitude. Lancet 362 Suppl: s16-s17.
- Cable GG (2003) In-flight hypoxia incidents in military aircraft: causes and implications for training. Aviat Space Environ Med 74(2): 169-172.
- Files DS, Webb JT, Pilmanis AA (2005) Depressurization in military aircraft: rates, rapidity, and health effects for 1055 incidents. Aviat Space Environ Med 76(6): 523-529.
- Chawla A, Lavania AK (2001) Oxygen toxicity. Med J Armed Forces India 57(2): 131-133.
- Maiti P, Singh SB, Sharma AK, Muthuraju S, Banerjee PK, et al. (2006) Hypobaric hypoxia induces oxidative stress in rat brain. Neurochem Int 49(8): 709-716.
- Onodera K, Omoi NO, Fukui K, Hayasaka T, Shinkai T, et al. (2003) Oxidative damage of rat cerebral cortex and hippocampus, and changes in antioxidative defense systems caused by hyperoxia. Free Radic Res 37(4): 367-372.
- Scholey AB, Moss MC, Neave N, Wesnes K (1999) Cognitive performance, hyperoxia, and heart rate following oxygen administration in healthy young adults. Physiol Behav 67(5): 783-789.
- Moss MC, Scholey AB, Wesnes K (1998) Oxygen administration selectively enhances cognitive performance in healthy young adults: a placebo-controlled double-blind crossover study. Psychopharmacology (Berl) 138(1): 27-33.
- Gozes I, Morimoto BH, Tiong J, Fox A, Sutherland K, et al. (2005) NAP: research and development of a peptide derived from activity-dependent neuroprotective protein (ADNP). CNS Drug Rev 11(4): 353-368.
- Shadrina M, Kolomin T, Agapova T, Agniullin Y, Shram S, et al. (2010) Comparison of the temporary dynamics of NGF and BDNF gene expression in rat hippocampus, frontal cortex, and retina under Semax action. J Mol Neurosci 41(1): 30-35.
- Medvedeva EV, Dmitrieva VG, Limborska SA, Myasoedov NF, Dergunova LV, et al. (2017) Semax, an analog of ACTH (4-7), regulates expression of immune response genes during ischemic brain injury in rats. Mol Genet Genomics 292(3): 635-653.
- Asmarin IP, Nezavibat'ko VN, Miasoedov NF, Kamenskiĭ AA, Grivennikov IA, et al. (1997) A nootropic adrenocorticotropin analog 4-10-semax (l5 years’ experience in its design and study). Zh Vyssh Nerv Deiat Im I P Pavlova 47(2): 420-430.
- Gozes I, Giladi E, Pinhasov A, Bardea A, Brenneman DE, et al. (2000) Activity-dependent neurotrophic factor: intranasal administration of femtomolar-acting peptides improve performance in a water maze. J Pharmacol Exp Ther 293(3):1091-1098.
- Barnes AK, Smith SB, Datta S (2017) Beyond Emotional and Spatial Processes: Cognitive Dysfunction in a Depressive Phenotype Produced by Long Photoperiod Exposure. PLoS One 12(1): e0170032.
- Inostroza M, Binder S, Born J (2013) Sleep-dependency of episodic-like memory consolidation in rats. Behav Brain Res 237: 15-22.
- Robertson GL, Mahr EA, Athar S, Sinha T (1973) Development and clinical application of a new method for the radioimmunoassay of arginine vasopressin in human plasma. J Clin Invest 52(9): 2340-2352.
- Preibisz JJ, Sealey JE, Laragh JH, Cody RJ, Weksler BB (1983) Plasma and platelet vasopressin in essential hypertension and congestive heart failure. Hypertension 5(2 Pt 2): I129-I138.
- Oltmanns KM, Gehring H, Rudolf S, Schultes B, Hackenberg C, et al. (2006) Acute hypoxia decreases plasma VEGF concentration in healthy humans. Am J Physiol Endocrinol Metab 290(3): E434-E439.
- Molina F, Rus A, Peinado MA, Del Moral ML (2013) Short-term hypoxia/reoxygenation activates the angiogenic pathway in rat caudate putamen. J Biosci 38(2): 363-371.
- Maslova MV, Maklakova AS, Sokolova NA, Ashmarin IP, Goncharenko EN, et al. (2003) The effects of ante- and postnatal hypoxia on the central nervous system and their correction with peptide hormones. Neurosci Behav Physiol 33(6): 607-611.
- Maslova MV, Graf AV, Samoilenkova NS, Maklakova AS, Sokolova NA, et al. (2003) Consequences of progestational hypoxia in pregnant rats and its peptide correction (EEG data). Bull Exp Biol Med 135(6): 526-529.
- Maslova MV, Graf AV, Sokolova NA, Goncharenko EN, Shestakova SV, et al. (2003) Effect of acute progestational hypoxia on the content of biogenic amines in the brain of albino rat pups: Peptide correction. Bull Exp Biol Med 136(2): 123-125.
- Silachev DN, Shram SI, Shakova FM, Romanova GA, Myasoedov NF, et al. (2009) Formation of spatial memory in rats with ischemic lesions to the prefrontal cortex; effects of a synthetic analog of ACTH (4-7). Neurosci Behav Physiol 39(8): 749-756.
- Rotstein M, Bassan H, Kariv N, Speiser Z, Harel S, et al. (2006) NAP enhances neurodevelopment of newborn apolipoprotein E-deficient mice subjected to hypoxia. J Pharmacol Exp Ther 319(1): 332-339.
- Greggio S, Rosa RM, Dolganov A, de Oliveira IM, Menegat FD, et al. (2009) NAP prevents hippocampal oxidative damage in neonatal rats subjected to hypoxia-induced seizures. Neurobiol Dis 36(3): 435-444.
- Greggio S, de Paula S, de Oliveira IM, Trindade C, Rosa RM, et al. (2011) NAP prevents acute cerebral oxidative stress and protects against long-term brain injury and cognitive impairment in a model of neonatal hypoxia-ischemia. Neurobiol Dis 44(1): 152-159.
- Sharma NK, Sethy NK, Meena RN, Ilavazhagan G, Das M, et al. (2011)tivity-dependent neuroprotective protein (ADNP)-derived peptide (NAP) ameliorates hypobaric hypoxia induced oxidative stress in rat brain. Peptides 32(6): 1217-1224.
- Zhao M, Cheng X, Lin X, Han Y, Zhou Y, et al. (2019) Metformin administration prevents memory impairment induced by hypobaric hypoxia in rats. Behav Brain Res 363: 30-37.
- Chauhan G, Roy K, Kumar G, Kumari P, Alam S, et al. (2019) Distinct influence of COX-1 and COX-2 on neuroinflammatory response and associated cognitive deficits during high altitude hypoxia. Neuropharmacology 146: 138-148.
- Zhu M, Xu M, Zhang K, Li J, Ma H, et al. (2019) Effect of acute exposure to hypobaric hypoxia on learning and memory in adult Sprague-Dawley rats. Behav Brain Res 367: 82-90.
- Zhang XY, Zhang XJ, Xv J, Jia W, Pu XY, et al. (2018) Crocin attenuates acute hypobaric hypoxia-induced cognitive deficits of rats. Eur J Pharmacol 818: 300-305.
- Kandikattu HK, Deep SN, Razack S, Amruta N, Prasad D, et al. (2017) Hypoxia induced cognitive impairment modulating activity of Cyperus rotundus. Physiol Behav 175: 56-65.
- Liu P, Zou D, Yi L, Chen M, Gao Y, et al. (2015) Quercetin ameliorates hypobaric hypoxia-induced memory impairment through mitochondrial and neuron function adaptation via the PGC-1α Restor Neurol Neurosci 33(2): 143-157.
- Shukitt Hale B, Stillman MJ, Lieberman HR (1996) Tyrosine administration prevents hypoxia-induced decrements in learning and memory. Physiol Behav 59(4-5): 867-871.
- Li W, Yang S, Yu FY, Zhao Y, Sun ZM, et al. (2018) Hydrogen ameliorates chronic intermittent hypoxia-induced neurocognitive impairment via inhibiting oxidative stress. Brain Res Bull 143: 225-233.
- Gao H, Han Z, Huang S, Bai R, Ge X, et al. (2017) intermittent hypoxia caused cognitive dysfunction relate to miRNAs dysregulation in hippocampus. Behav Brain Res 335: 80-87.
- Das S, Mishra KP, Ganju L, Singh SB (2018) Intranasally delivered small interfering RNA-mediated suppression of scavenger receptor Mac-1 attenuates microglial phenotype switching and working memory impairment following hypoxia. Neuropharmacology 137: 240-255.
- Koester Hegmann C, Bengoetxea H, Kosenkov D, Thiersch M, Haider T, et al. (2019) Schneider Gasser EM. High-Altitude Cognitive Impairment Is Prevented by Enriched Environment Including Exercise via VEGF Signaling. Front Cell Neurosci 12: 532.
- Beni Adani L, Gozes I, Cohen Y, Assaf Y, et al. (2001) A peptide derived from activity-dependent neuroprotective protein (ADNP) ameliorates injury response in closed head injury in mice. J Pharmacol Exp Ther 296(1): 57-63.
- Kumral A, Yesilirmak DC, Sonmez U, Baskin H, Tugyan K, et al. (2006) Neuroprotective effect of the peptides ADNF-9 and NAP on hypoxic-ischemic brain injury in neonatal rats. Brain Res 1115(1): 169-178.
- Leker RR, Teichner A, Grigoriadis N, Ovadia H, Brenneman DE, et al. (2002) NAP, a femtomolar-acting peptide, protects the brain against ischemic injury by reducing apoptotic death. Stroke 33(4): 1085-1092.
- Zhang J, Wei SY, Yuan L, Kong LL, Zhang SX, et al. (2017) Davunetide improves spatial learning and memory in Alzheimer's disease-associated rats. Physiol Behav 174: 67-73.
- Gozes I, Alcalay R, Giladi E, Pinhasov A, Furman S, et al. (2002) NAP accelerates the performance of normal rats in the water maze. J Mol Neurosci 19(1-2): 167-170.
- Romanova GA, Silachev DN, Shakova FM, Kvashennikova YN, Viktorov IV, et al. (2006) Neuroprotective and antiamnesic effects of Semax during experimental ischemic infarction of the cerebral cortex. Bull Exp Biol Med 142(6): 663-666.
- Malyshev AV, Razumkina EV, Dubynin VA, Myasoedov NF (2013) Semax corrects brain dysfunction caused by prenatal introduction of valproic acid. Dokl Biol Sci 450: 126-129.
- Maslova MV, Zemlyanskii KS, Shkol'nikova MV, Maklakova AS, Krushinskaya YV, et al. (2001)Peptidergic correction of the effect of acute hypobaric hypoxia in pregnant rats on progeny. Bull Exp Biol Med 131(2): 109-112.
- Inozemtsev AN, Bokieva SB, Karpukhina OV, Gumargalieva KZ, Kamensky AA, et al. (2016) Semax prevents learning and memory inhibition by heavy metals. Dokl Biol Sci 468(1): 112-114.
- Inozemtsev AN, Agapitova AE, Bokieva SB, Levitskaya NG, Kamenskii AA, et al. (2013) Semax prevents functional impairments of avoidance response in rats. Dokl Biol Sci 448: 1-3.
- Ramanantsoa N, Fleiss B, Bouslama M, Matrot B, Schwendimann L, et al. (2013) Bench to cribside: the path for developing a neuroprotectant. Transl Stroke Res 4(2): 258-277.
- Hassell KJ, Ezzati M, Alonso Alconada D, Hausenloy DJ, Robertson NJ (2015) New horizons for newborn brain protection: enhancing endogenous neuroprotection. Arch Dis Child Fetal Neonatal Ed 100(6): F541-F542.
- Ziemka Nalecz M, Jaworska J, Sypecka J, Polowy R, Filipkowski RK, et al. (2017) Sodium Butyrate, a Histone Deacetylase Inhibitor, Exhibits Neuroprotective/Neurogenic Effects in a Rat Model of Neonatal Hypoxia-Ischemia. Mol Neurobiol 54(7): 5300-5318.
- Zhou H, Wang J, Jiang J, Stavrovskaya IG, Li M, et al. (2014) N-acetyl-serotonin offers neuroprotection through inhibiting mitochondrial death pathways and autophagic activation in experimental models of ischemic injury. J Neurosci 34(8): 2967-2978.
- Luo C, Yang Q, Liu Y, Zhou S, Jiang J, et al. (2019) The multiple protective roles and molecular mechanisms of melatonin and its precursor N-acetylserotonin in targeting brain injury and liver damage and in maintaining bone health. Free Radic Biol Med 130: 215-233.
- Pazos MR, Cinquina V, Gómez A, Layunta R, Santos M, et al. (2012) Cannabidiol administration after hypoxia-ischemia to newborn rats reduces long-term brain injury and restores neurobehavioral function. Neuropharmacology 63(5): 776-783.
- Chung H, Seo S, Moon M, Park S (2008) Phosphatidylinositol-3-kinase/Akt/glycogen synthase kinase-3 beta and ERK1/2 pathways mediate protective effects of acylated and unacylated ghrelin against oxygen-glucose deprivation-induced apoptosis in primary rat cortical neuronal cells. J Endocrinol 198(3): 511-521.
- Chung H, Choi J, Park S (2018) Ghrelin protects adult rat hippocampal neural stem cells from excessive autophagy during oxygen-glucose deprivation. Endocr J 65(1): 63-73.
- Minciu Macrea M, Misra H, Zagrean L (2010) the neuroprotective effect of intranasally applied leptin against hypoxic neuronal injury. Med Hypotheses 74(6): 1036-1037.
- Chen A, Xiong LJ, Tong Y, Mao M (2013) The neuroprotective roles of BDNF in hypoxic ischemic brain injury. Biomed Rep 1(2): 167-176.
- Bolouri H, Sävman K, Wang W, Thomas A, Maurer N, et al. (2014) Innate defense regulator peptide 1018 protects against perinatal brain injury. Ann Neurol 75(3): 395-410.
- Li F, Tan J, Zhou F, Hu Z, Yang B, et al. (2018) Heat Shock Protein B8 (HSPB8) Reduces Oxygen-Glucose Deprivation/Reperfusion Injury via the Induction of Mitophagy. Cell Physiol Biochem 48(4): 1492-1504.
- Yang D, Kuan CY (2015) Anti-tissue plasminogen activator (tPA) as an effective therapy of neonatal hypoxia-ischemia with and without inflammation. CNS Neurosci Ther 21(4): 367-373.
- Xiong S, Xu Y, Ma M, Wang H, Wei F, et al. (2017) Neuroprotective effects of a novel peptide, FK18, under oxygen-glucose deprivation in SH-SY5Y cells and retinal ischemia in rats via the Akt pathway. Neurochem Int 108: 78-90.
- Vaczy A, Kovari P, Kovacs K, Farkas K, Szabo E, et al. (2018) Protective Role of Endogenous PACAP in Inflammation-induced Retinal Degeneration. Curr Pharm Des 24(30): 3534-3542.
- Stankowska DL, Nam MH, Nahomi RB, Chaphalkar RM, Nandi SK, et al. (2019) Systemically administered peptain-1 inhibits retinal ganglion cell death in animal models: implications for neuroprotection in glaucoma. Cell Death Discov 5: 122.
- Edwards AB, Anderton RS, Knuckey NW, Meloni BP (2018) Perinatal Hypoxic-Ischemic Encephalopathy and Neuroprotective Peptide Therapies: A Case for Cationic Arginine-Rich Peptides (CARPs). Brain Sci 8(8): E147.
- Burton VJ, Gerner G, Cristofalo E, Chung SE, Jennings JM, et al. (2015) A pilot cohort study of cerebral autoregulation and 2-year neurodevelopmental outcomes in neonates with hypoxic-ischemic encephalopathy who received therapeutic hypothermia. BMC Neurol 15: 209.
- Massaro AN, Evangelou I, Fatemi A, Vezina G, Mccarter R, et al. (2015) White matter tract integrity and developmental outcome in newborn infants with hypoxic-ischemic encephalopathy treated with hypothermia. Dev Med Child Neurol 57(5): 441-448.
- Reinboth BS, Köster C, Abberger H, Prager S, Bendix I, et al. (2016) Endogenous hypothermic response to hypoxia reduces brain injury: Implications for modeling hypoxic-ischemic encephalopathy and therapeutic hypothermia in neonatal mice. Exp Neurol 283(Pt A): 264-275.
- Teo JD, Morris MJ, Jones NM (2017) Hypoxic postconditioning improves behavioural deficits at 6 weeks following hypoxic-ischemic brain injury in neonatal rats. Behav Brain Res 333: 27-34.
- Pereira LO, Strapasson AC, Nabinger PM, Achaval M, Netto CA, et al. (2008) Early enriched housing results in partial recovery of memory deficits in female, but not in male, rats after neonatal hypoxia-ischemia. Brain Res 1218: 257-266.
- Nguyen AQ, Cherry BH, Scott GF, Ryou MG, Mallet RT, et al. (2014) Erythropoietin: powerful protection of ischemic and post-ischemic brain. Exp Biol Med (Maywood) 239(11): 1461-1475.
- Juul S (2002) Erythropoietin in the central nervous system, and its use to prevent hypoxic-ischemic brain damage. Acta Paediatr Suppl 91(438): 36-42.
- Nekoui A, Blaise G (2017) Erythropoietin and Nonhematopoietic Effects. Am J Med Sci353(1): 76-81.
- Lippi G, Franchini M, Favaloro EJ (2010) Thrombotic complications of erythropoiesis-stimulating agents. Semin Thromb Hemost 36: 537-549.
- O'Shaughnessy JA (2002) Effects of epoetin alfa on cognitive function, mood, asthenia, and quality of life in women with breast cancer undergoing adjuvant chemotherapy. Clin Breast Cancer 3 Suppl 3: S116-S120.
- Xiong T, Yang X, Qu Y, Chen H, Yue Y, et al. (2019) Erythropoietin induces synaptogenesis and neurite repair after hypoxia ischemia-mediated brain injury in neonatal rats. Neuroreport 30(11): 783-789.
- Melincovici CS, Boşca AB, Şuşman S, Mărginean M, Mihu C, et al. (2018) Vascular endothelial growth factor (VEGF) - key factor in normal and pathological angiogenesis. Rom J Morphol Embryol 59(2): 455-467.
- Siebenmann C, Cathomen A, Hug M, Keiser S, Lundby AK, et al. (2005) Rasmussen P, Lundby C. Hemoglobin mass and intravascular volume kinetics during and after exposure to 3,454-m altitude. J Appl Physiol 119(10): 1194-1201.
- Kiboub FZ, Møllerløkken A, Hjelde A, Flatberg A, Loennechen Ø, et al. (2018) Blood Gene Expression and Vascular Function Biomarkers in Professional Saturation Diving. Front Physiol 9: 937.
- Zhang Y, Singh I, Krzyzanski W (2014) Dynamics of Erythropoietic Markers in Response to Hypoxia in Rats. J Blood Disorders Transf 5(3): 197-204.
- Chowdhury A, Dasgupta R (2017) Effects of acute hypoxic exposure on oxygen affinity of human red blood cells. Appl Opt 56(3): 439-445.
- Atlas SA (2007) the renin-angiotensin aldosterone system: pathophysiological role and pharmacologic inhibition. J Manag Care Pharm 13(8 Suppl B): 9-20.
- Marchi N, Cavaglia M, Fazio V, Bhudia S, Hallene K, et al. (2004) Peripheral markers of blood–brain barrier damage. Clin Chim Acta 342(1-2): 1-12.
- Czeisler BM, Janigro D (2006) Reading and writing the blood–brain barrier: relevance to therapeutics. Recent Pat CNS Drug Discov 1(2): 157-173.
- Savourey G, Launay JC, Besnard Y, Guinet A, Travers S, et al. (2003) Normo- and hypobaric hypoxia: are there any physiological differences? Eur J Appl Physiol 89(2): 122-126.
- Faiss R, Pialoux V, Sartori C, Faes C, Dériaz O, et al. (2013) Ventilation, oxidative stress, and nitric oxide in hypobaric versus normobaric hypoxia. Med Sci Sports Exerc 45(2): 253-260.
- Self DA, Mandella JG, Prinzo OV, Forster EM, Shaffstall RM, et al. (2011) Physiological equivalence of normobaric and hypobaric exposures of humans to 25,000 feet (7620 m). Aviat Space Environ Med 82(2): 97-103.
- Malle C, Bourrilhon C, Quinette P, Laisney M, Eustache F, et al. (2016) Physiological and Cognitive Effects of Acute Normobaric Hypoxia and Modulations from Oxygen Breathing. Aerosp Med Hum Perform 87(1): 3-12.