Mini Review
Creative Commons, CC-BY
Applications of FRET-Based Supramolecular Architectures for Temperature Sensing and Cancer Diagnosis: A Mini-Review
*Corresponding author: Amir Sohail, Chemistry Department, College of Science, United Arab Emirates University, United Arab Emirates
Received: October 22, 2021; Published: November 09, 2021
DOI: 10.34297/AJBSR.2021.14.002031
Abstract
Supramolecular nanostructured materials, displaying Forster Resonance Energy Transfers (FRET) signals, have become the focus of interest for many researchers across the globe. FRET based supramolecular systems have extended applications in areas as diverse as materials science, biochemistry, analytical chemistry, and nanomedicine. The non-covalent phenomena operating in supramolecular frameworks depends on many factors such as wide range of time scales, binding strengths, distances, and concentrations of the supramolecular components (host and guest). Here in, we focus on which FRET has been used to study non-covalent interactions having a key role of cancer diagnosis and temperature sensing in supramolecular systems. Furthermore, we have discussed FRET-based architectures with current advancement in the field and provide a perspective on new advancement for the future.
Keywords: Supramolecular Architectures, Forster Resonance Energy Transfers, Non-Covalent Interactions, Host-Guest Chemistry, FRET Signals, Supramolecular, Hydrophobic Forces, Electrostatic Interactions, Hydrogen Bonding, Van Der Waals Forces, Chemical Systems
Introduction
Supramolecular chemistry describes chemical systems composed of a discrete number of molecules with self-organization tendency through weak and reversible non-covalent interaction. Supramolecular science is heavily relying on macrocycle-based host-guest chemistry [1,2]. Typically, in a host-guest system, a large macrocyclic host recognize a small guest molecule, binds to each other through non-covalent interactions (hydrophobic forces, electrostatic interactions, hydrogen bonding, Van der Waals forces) in a controlled manner [3]. This binding of host and guest molecule leads to the formation of host-guest complex [1,3,4] More importantly, the guest molecules should fit in the cavity of host molecules, emphasizing on selective and spontaneous binding of host and guest, and thus realizing the molecular recognition [5-7]. Host-guest molecular recognition has brought revolutions in the development of more sophisticated supramolecular systems or materials because of their good selectivity and stimuli responsiveness.
Taking advantage of weaker non-covalent host-guest interactions, the host-guest complexes are more suitable for nice reversibility and responsiveness towards various factors in the confined surrounding environment such as pH, light radiation, temperature, competitive factors, chemical signals, and biological interfering, etc [3,4,6-8]. The stimuli-responsive property of host-guest systems provide a favorable platform for the developing of new advanced artificial molecular machines and nanoscale smart material [9-11]. In the past decades, many FRET based supramolecular system has been reported with extended applications such as optical sensor, photo-switcher, artificial light harvesting system etc [12-15]. FRET got attention of many researchers as it the concept of FRET was first disclosed by Theodor Forster with a remarkable discovery of Green fluorescent Proteins (GFPs) [16] FRET based approaches are considered applicable in the diagnosis of cancer, temperature sensing due to its high sensitivity, versatility, and non-invasiveness [17-19].
Forster provide the basis of quantitative description for the non-radiative energy transfer between the donor and acceptor molecules. Practically, in a FRET phenomenon, a donor fluorophore after absorption of light energy is excited to high energy state which in turn release the absorbed energy as emission of light, transfer its energy non-radiatively to the nearby acceptor fluorophore [20] Moreover, the excitation energy of the donor’s electron is transferred to that of the acceptors via an induced-dipole movement interaction [21]. FRET is a more powerful tool than simple fluorescence because it is very sensitive to small changes in the ambient environment. There are many conditions that need to be met for the occurrence of FRET phenomena. Firstly, to ensure the occurrence of FRET, the process require efficient spectral overlap of donor emission with acceptor fluorophore absorption considering molecular electronic excitation and energy release [1,22,23].
Secondly, the donor and acceptor fluorophores should be at favorable distance and needs to be less than approximately 10nm [24]. The third and final criteria for the efficient energy transfer are the proper orientation of both the fluorophores to each other. Once all the conditions are satisfied, the FRET efficiency [25] and thus relying on the number of photons absorbed by donor fluorophores that has a key role in contribution of FRET and can provide highly sensitive, temporally specific information on molecular distance and orientation These features broaden the scope of FRET and well suited to study a wide range of supramolecular phenomena with extended application in many fields. Moreover, the distance between the donor and acceptor as given by Equation (1).

Where energy transfer efficiency is represented by E and r stands for the donor-acceptor distance. R0 is the Forster radiusvaries with the size of donor-acceptor pairs and depends spectral overlap. In general, three types of fluorescent activities have been noted in a FRET phenomenon, namely, the turn-off effect on the donor, the switch-on fluorescence of the acceptor and, sometimes, when the acceptor possesses fluorescence quenching abilityquench the emission of donor and acceptor. Surprisingly, the functioning of FRET inside the host-guest system facilitates donoracceptor pair association and dissociation with flexibility and controllability due to the stimuli-responsiveness and selectivity exhibited by host-guest non-covalent interactions.
The combination of FRET with host guest science possesses more advanced applications in many fields such as real-time in vivo monitoring of biomolecules, and structural manipulation [26,27] cell imaging and drug delivery [28,29] chemical and biological sensing [30-33] and photosynthesis mimicking [18,34-36] etc. Here in, we particularly focus on FRET operating supramolecular systems used for the diagnosis of cancer, and temperature sensing. To ensure the current developments in the field, an up-to-date literature study was carried for signifying our understanding of cellular processes and help in advanced approaches applied in synthetic supramolecular systems and their in vivo applications.
Applications of supramolecular FRET System Based on Host-Guest Chemistry
Temperature Sensing
Very recently, Amir et al were successful in preparing a bioactive, supramolecular carbohydrate polymer by implanting Cucurbit [7] uril macrocycle (CB7)-encapsulated Dequalinium Chloride Hydrate (DCH) onto Alginic Acid Carbohydrates (ALG) via amide linkage formation having the capability of light energy transfer heavily relying on energy migration can be controlled by varying polymer temperature without changing the polymer [4] (Figure 1). Schematic representation of supramolecular carbohydrates polymer synthesis and modulation of FRET signals by altering temperature [4]. Forster Resonance Energy Transfer (FRET) signals were generated using DCH (donor) and 2-anilinonaphthalene-6- sulfonic acid (acceptor). The modified carbohydrate platform’s stationery and time-resolved photoluminescence spectra indicated that FRET resulted in a color change from violet (387nm) to blue (429nm), which could be switched on and off to temperature stimuli at 298-368K. DCH alginate polymer was prepared by covalent linkage of DCH to alginic acid (via amide linkage) with/ without CB7.
In the first step, alginic acid is activated in DMSO with N, N′- Dicyclohexylcarbodiimide (DCC), 4-Dimethylaminopyridinefor (DMP) used as coupling reagent for 24 hours under constant nitrogen purging (as depicted in Figure 1) (Figure 2). To validate energy transfer, DCHALG and DCH/CB7ALG polymer was titrated with ANS. No FRET signals were observed as no significant decrease in the excited lifetime was recorded while titrating DCHALG with ANS as shown in figure 2a and 2c. To confirm FRET signals occurrence in DCH/CB7ALG upon titration with ANS, a substantial decrease in the excited state was recorded and thus confirm the FRET signals between DCH/CB7ALG and ANS as depicted in figure 2b and 2d. Prior to any emission signal measurements, we first began energy transfer by introducing 0.5μM ANS at 298K to guarantee the integrity of our examination. Following that, the temperature was reduced to 278 K, increased to 378K, and then returned to 278K. After ANS addition, the process was repeated three times with an ANS concentration rise of 2.75M every cycle (up to 8.75M).
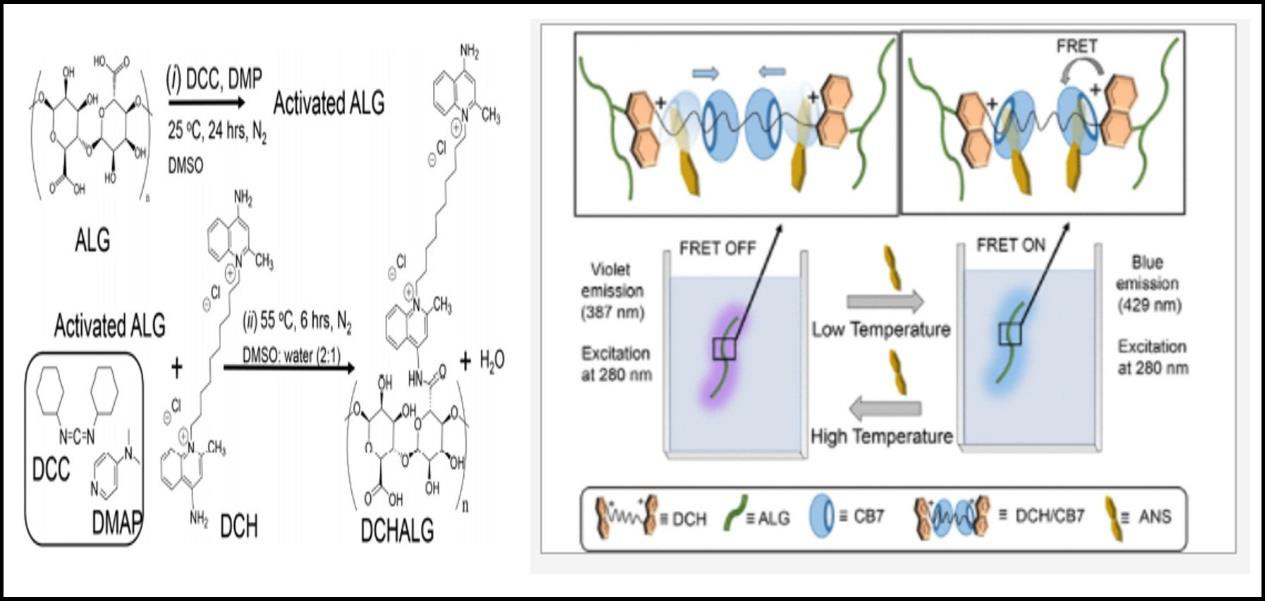
Figure 1: Schematic representation of supramolecular carbohydrates polymer synthesis and modulation of FRET signals by altering temperature.
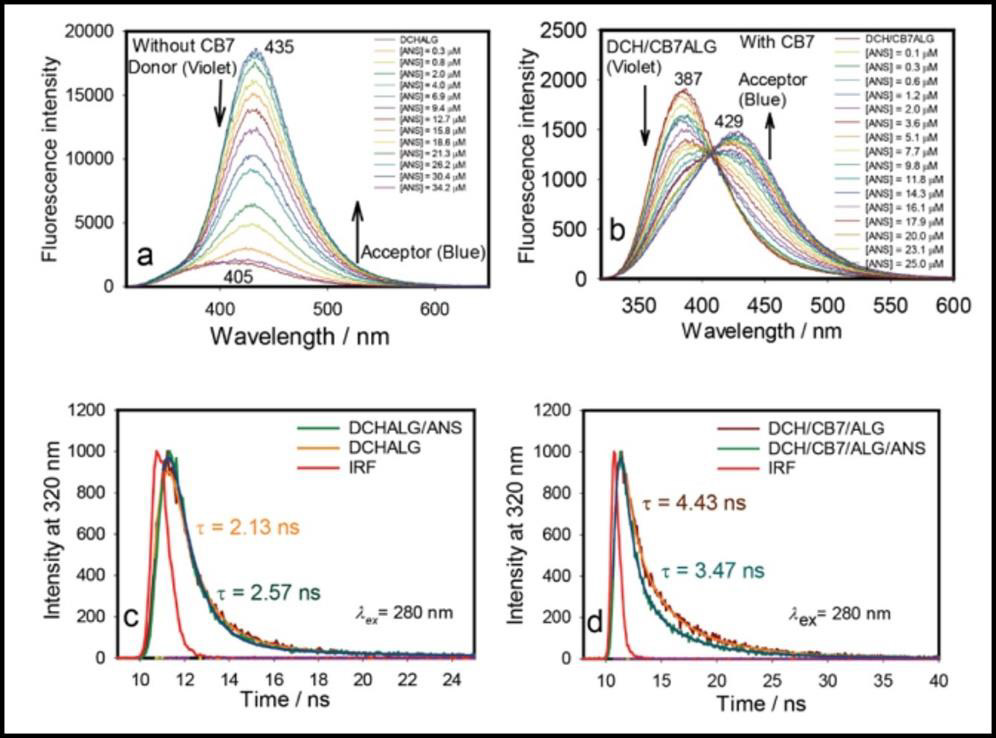
Figure 2: At various concentrations, energy transfer from (a) DCHALG (1.5 mg L-1 aqueous suspension) and (b) DCH/CB7ALG (2.75 mg mL-1 aqueous suspension) to ANS. Corresponding 320 nm emission decays indicated by the estimated average excited-state lifetime in the absence and presence of CB7 (c) and (d).
We used the technique shown in Figure 3 to obtain emission data. The results in Figure 3 demonstrate the reversible response of DCH/CB7ALG to a temperature change from 278 to 378 K after adding an incremental quantity of ANS (7M, from 8.75 to 33.3M) four times to create five sensing cycles. The results validated the novel supramolecular polymers’ response to temperature stimuli at 298-368K in aqueous environments. It should be emphasized that in Figure 3, the unquenched/quenched lifespan ratio (τo/τ) was determined in each cycle by simply changing the temperature from 298 to 368 K. Furthermore, we assessed the excited-state lifetime rather than the steady-state spectrum since the former was more suitable for validating FRET signal modulation in response to a thermal change. Furthermore, it can be assumed that the proposed supramolecular polymers are nontoxic and physiologically active, and hence have a wide range of biomedical uses in the future.
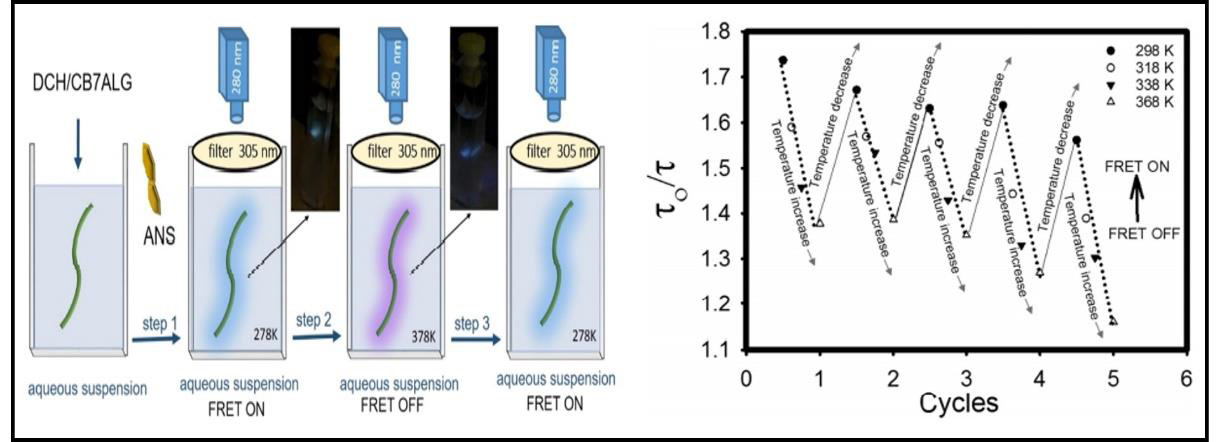
Figure 3: Schematic representation of FRET signal regeneration in aqueous suspensions and FRET signal on/off switching between 298 and 368 K in 20 K increments was monitored by the rise in the unquenched/quenched lifespan ratio following temperature reduction (FRET ON) and increase in temperature (FRET OFF) .
Cancer Diagnosis
Wang and colleagues demonstrate in their research a supramolecular fluorescent nanoparticle retying on FRET for detection of hydrogen peroxide (H2O2) in cancer infected cells [37]. Utilizing the FRET signal, a Supramolecular fluorescent nanoparticle self-assembled from fluorescein isothiocyanate modified β-cyclodextrin (FITC-β-CD)/Rhodamine B Modified Ferrocene (Fc-RB) amphiphile were employed for H2O2 detection in cancer cells as depicted in Figure 4. The self-assembled nanoparticles based on numerous non-covalent contacts in aqueous medium demonstrated great sensitivity to H2O2 while being stable under physiological conditions. The addition of H2O2 resulted in a noticeable fluorescence shift of nanoparticles from Red (RB) to Green (FITC) in fluorescent tests due to the Fluorescence Resonance Energy Transfer (FRET) phenomenon. Furthermore, in vitro results clearly indicate that fluorescent nanoparticles may be effectively absorbed by cancer cells and then destroyed by endogenous H2O2, accompanied by FRET from “on” to “off.” These supramolecular fluorescent nanoparticles, which were created through a series of non-covalent interactions, are likely to have applications in the diagnosis and imaging of illnesses induced by oxidative stress.
For many years, nanocarriers have been particularly designed for the transport and controlled release of drugs and biological molecules. However, cargo loading and release of frequently utilized carriers are difficult to monitor, particularly in vivo. Huang and colleagues described in their study, a [2] rotaxane complexation with pillar [5] arene (P5) to achieve mitochondria imaging and drug delivery materials [28]. In this work, Aggregation-Induced Emission (AIE) and Aggregation-Caused Quenching (ACQ) were introduced in a host-guest controlled FRET investigation. A [2] rotaxane with increased fluorescence was produced by attaching a Tetraphenyl Ethylene (TPE) unit, a typical AIE-active luminophore, as a stopper on one end of the axle with pillar [5] arene acting as the wheel. The anticancer medication Doxorubicin (DOX), as well as the ACQ agent, were covalently attached to the wheel through imine bridges and therefore positioned near to TPE, resulting in a significant failure of the fluorescence emission owing to the ACQ effect, which happened during FRET from TPE to DOX.
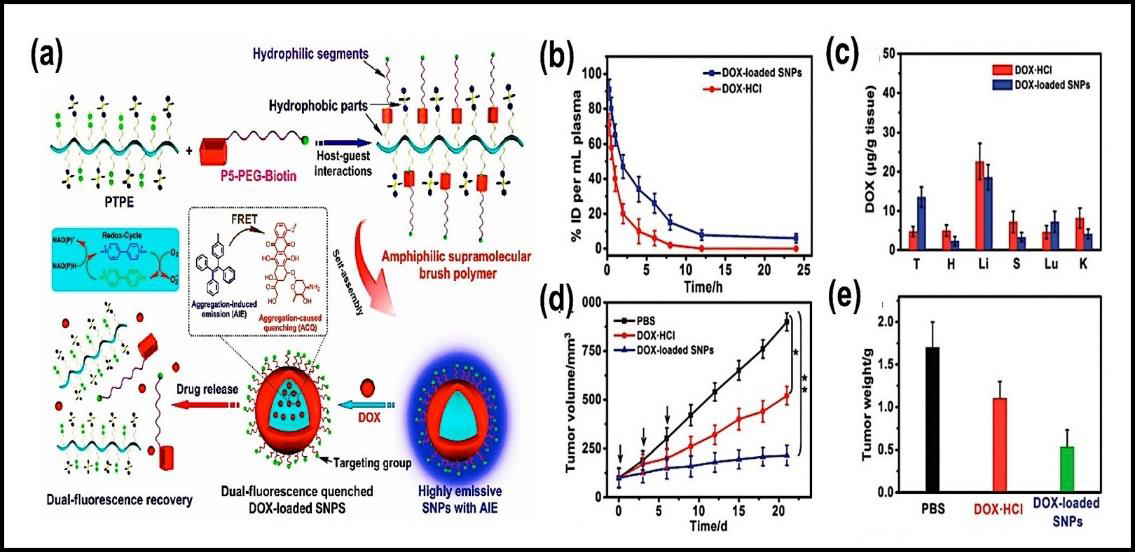
Figure 5: (a) Procedure for the creation and disassembly of SNPs with DOX loading and dual fluorescence quenching; (b–e) Graphical depiction of the drug-delivering SNPs’ in vivo effects: (b) blood circulation time of DOXHCl and DOX-loaded SNPs as measured by DOX plasma concentration after injection; (d) Tumor growth inhibition curves on the HeLa tumor model treated with phosphate buffered saline (PBS), DOXHCl, and DOXloaded SNPs, in that order. (e) The average weight of tumors in mice carrying HeLa tumors following the three different treatments indicated above. 2016. Copyright. The Royal Society of Chemistry has granted permission for this reprint.
Because of the additional negative membrane potential of mitochondria, it may be identified by the [2] rotaxane via electrostatic interactions and lighted up because of the recognition. Based on the above foundation, they started to create FRET-capable SNPs for DOX delivery that were constructed using pillar [5] arenebased amphyphilic Supramolecular Brush Copolymers (SBPs)29 TPE and 4,4′-bipyridinium derivative (M) moieties were alternately implanted onto the polymer. The host-guest interactions formed between the M entities and PEG-Biotin (targeting group), as illustrated in Figure 5a, functionalized P5. As a result, SBPs were produced and then self-assembled into SNPs, which displayed an AIE effect caused by the aggregation of TPE units in the particles’ center. The system’s emission decreased when DOX was encapsulated in the SNPs because to the FRET from TPE to DOX and the ACQ of the DOX units, resulting in dual-fluorescence quenching.
When the guest M was reduced by the intracellular reductase NAD(P)H in an acidic environment from a bicationic entity to its radical cationic state, the binding between them and P5 was severely weakened, with the association constant dwindling by two orders of magnitude, resulting in the detachment of the host-guest pair and the disassociation of the SNPs. DOX was therefore liberated from the confinement of the particles and leads to the restoration of fluorescence as well. Figure 5b-e demonstrates the controlled drug releasing characteristics, distributions in normal tissues, and cancer cell inhibition efficiency, indicating the good encapsulation and cancer targeting capabilities (DOX concentration was higher in tumor treated with DOX-loaded SNPs than with DOX alone in contrast to that in other organs). In this work, the proximity/ separation of the FRET pair was regulated in a very circuitous, but subtle, way by modulating the assembly of SBPs and FRET at the same time, producing an exquisite collaboration between stimuliresponsiveness of host-guest chemistry and fluorescence signaling of FRET.
Conclusion and Future Perspectives
Keeping in mind the fact that the innovative collaboration between FRET effects and host-guest chemistry has bring significant changes in the fabrication of a wide range of smart fluorescent materials with stimuli-responsive properties. However, in this emerging field, there are still many for researchers to overcome to play better light tricks. These supramolecular fluorescent nanoparticles, which were created through a noncovalent interaction, are likely to have applications in the diagnosis of cancer disease and temperature sensing. Moreover, the amazing evolution of dynamically constructed FRET systems backed by supramolecular macrocyclic chemistry has resulted in the creation of a wide range of smart fluorescent materials with extended applications. Furthermore, it is believed that more advanced supramolecular frameworks will have numerous biomedical applications in the future.
Acknowledgement
All authors thank the research program in United Arab Emirates University.
Conflict of Interest
No conflict of interest.
References
- Lou XY, Song N, Yang YW (2017) Fluorescence Resonance Energy Transfer Systems in Supramolecular Macrocyclic Chemistry. Molecules 22(10): 1640.
- Yao Q, Lü B, Ji C, Cai Y, Yin M, et al. (2017) Supramolecular Host-Guest System as Ratiometric Fe3+ Ion Sensor Based on Water-Soluble Pillar [5] Arene. ACS Appl Mater Interfaces 9(41): 36320-36326.
- Qu DH, Wang QC, Zhang QW, Ma X, Tian H, et al. (2015) Photoresponsive Host-Guest Functional Systems. Chem Rev 115(15): 7543-7588.
- Sohail A, Alnaqbi MA, Saleh N (2019) Alginate/Cucurbit [7] Uril/Dequalinium-Based Supramolecular Carbohydrates: Modulation of FRET Signals by Temperature Control. Macromolecules 52(22): 9023-9031.
- Hu J, Liu S (2014) Engineering Responsive Polymer Building Blocks with Host-Guest Molecular Recognition for Functional Applications. Acc Chem Res 47(7): 2084-2095.
- Liu Z, Nalluri SM, Stoddart JF (2017) Surveying Macrocyclic Chemistry: From Flexible Crown Ethers to Rigid Cyclophanes. Chem Soc Rev 46(9): 2459-2478.
- Yang YW, Sun YL, Song N (2014) Switchable Host-Guest Systems on Surfaces. Acc Chem Res 47(7): 1950-1960.
- Jones CD, Steed JW (2016) Gels with Sense: Supramolecular Materials That Respond to Heat, Light and Sound. Chem Soc Rev 45(23): 6546-6596.
- Cheng C, McGonigal PR, Schneebeli ST, Li H, Vermeulen NA, et al. (2015) An Artificial Molecular Pump. Nat Nanotechnol 10(6): 547-553.
- Klajn R, Stoddart JF, Grzybowski BA (2010) Nanoparticles Functionalized with Reversible Molecular and Supramolecular Switches. Chem Soc Rev 39(6): 2203-2237.
- Pitto-Barry A, Barry NP, Russo V, Heinrich B, Donnio B, et al. (2014) Designing Supramolecular Liquid-Crystalline Hybrids from Pyrenyl-Containing Dendrimers and Arene Ruthenium Metallacycles. J Am Chem Soc 136(50): 17616-17625.
- Qiao F, Zhang L, Lian Z, Yuan Z, Yan CY, et al. (2018) Construction of Artificial Light-Harvesting Systems in Aqueous Solution: Supramolecular Polymers Based on Host-Enhanced π-π Interaction with Aggregation-Induced Emission. J Photochem Photobiol Chem 355: 419-424.
- Wang P, Miao X, Meng Y, Wang Q, Wang J, et al. (2020) Tetraphenylethene-Based Supramolecular Coordination Frameworks with Aggregation-Induced Emission for an Artificial Light-Harvesting System. ACS Appl Mater Interfaces 12(20): 22630-22639.
- Hu Y, Li W, Jia P, Wang X, Xu L, et al. (2020) Supramolecular Artificial Light‐Harvesting Systems with Aggregation‐Induced Emission. Adv Opt Mater 8(14): 2000265.
- Bhattacharyya S, Maity M, Chowdhury A, Saha ML, Panja SK, et al. (2020) Coordination-Assisted Reversible Photoswitching of Spiropyran-Based Platinum Macrocycles. Inorg Chem 59(3): 2083-2091.
- Tsien RY (1998) THE GREEN FLUORESCENT PROTEIN. Annu Rev Biochem 67(1): 509-544.
- Severi C, Melnychuk N, Klymchenko AS (2020) Smartphone-Assisted Detection of Nucleic Acids by Light-Harvesting FRET-Based Nanoprobe. Biosens Bioelectron 168: 112515.
- Meng LB, Li D, Xiong S, Hu XY, Wang L, et al. (2015) FRET-Capable Supramolecular Polymers Based on a BODIPY-Bridged Pillar [5] Arene Dimer with BODIPY Guests for Mimicking the Light-Harvesting System of Natural Photosynthesis. Chem Commun 51(22): 4643-4646.
- Yao Y, Xue M, Chen J, Zhang M, Huang F, et al. (2012) An Amphiphilic Pillar [5] Arene: Synthesis, Controllable Self-Assembly in Water, and Application in Calcein Release and TNT Adsorption. J Am Chem Soc 134(38): 15712-15715.
- Chen NT, Cheng SH, Liu CP, Souris J, Chen CT, et al. (2012) Recent Advances in Nanoparticle-Based Forster Resonance Energy Transfer for Biosensing, Molecular Imaging and Drug Release Profiling. Int J Mol Sci 13(12): 16598-16623.
- Boisselier E, Astruc D (2009) Gold Nanoparticles in Nanomedicine: Preparations, Imaging, Diagnostics, Therapies and Toxicity. Chem Soc Rev 38(6): 1759-1782.
- Berney C, Danuser G (2003) FRET or No FRET: A Quantitative Comparison. Biophys J 84(6): 3992-4010.
- Sapsford KE, Berti L, Medintz IL (2006) Materials for Fluorescence Resonance Energy Transfer Analysis: Beyond Traditional Donor-Acceptor Combinations. Angew Chem Int Ed 45(28): 4562-4589.
- Bunt G, Wouters FS (2017) FRET from Single to Multiplexed Signaling Events. Biophys Rev 9(2): 119-129.
- Zhou D, Piper JD, Abell C, Klenerman D, Kang DJ, et al. (2005) Fluorescence Resonance Energy Transfer between a Quantum Dot Donor and a Dye Acceptor Attached to DNA. Chem Commun 38: 4807-4809.
- Nguyen HD, Dang DT, Van Dongen JL, Brunsveld L (2010) Protein Dimerization Induced by Supramolecular Interactions with Cucurbit [8] Uril. Angew Chem Int Ed 49(5): 895-898.
- Hossain MA, Mihara H, Ueno A (2003) Novel Peptides Bearing Pyrene and Coumarin Units with or without β-Cyclodextrin in Their Side Chains Exhibit Intramolecular Fluorescence Resonance Energy Transfer. J Am Chem Soc 125(37): 11178-11179.
- Yu G, Wu D, Li Y, Zhang Z, Shao L, et al. (2016) A Pillar [5] Arene-Based [2] Rotaxane Lights up Mitochondria. Chem Sci 7(5): 3017-3024.
- Yu G, Zhao R, Wu D, Zhang F, Shao L, et al. (2016) Pillar [5] Arene-Based Amphiphilic Supramolecular Brush Copolymers: Fabrication, Controllable Self-Assembly and Application in Self-Imaging Targeted Drug Delivery. Polym Chem 7(40): 6178-6188.
- Xu M, Wu S, Zeng F, Yu C (2010) Cyclodextrin Supramolecular Complex as a Water-Soluble Ratiometric Sensor for Ferric Ion Sensing. Langmuir 26(6): 4529-4534.
- Xue M, Wei W, Su Y, Johnson D, Heath JR, et al. (2016) Supramolecular Probes for Assessing Glutamine Uptake Enable Semi-Quantitative Metabolic Models in Single Cells. J Am Chem Soc 138(9): 3085-3093.
- Fu L, Lai G, Yu A (2015) Preparation of β-Cyclodextrin Functionalized Reduced Graphene Oxide: Application for Electrochemical Determination of Paracetamol. RSC Adv 5(94): 76973-76978.
- Ye H, Yang L, Zhao G, Zhang Y, Ran X, et al. (2016) A FRET-Based Fluorescent Approach for Labetalol Sensing Using Calix [6] Arene Functionalized MnO2 @graphene as a Receptor. RSC Adv 6(83): 79350-79360.
- Villafiorita MF, Daita V, Quarti C, Perdicchia D, Del Buttero P, et al. (2014) Light Harvesting of CdSe/CdS Quantum Dots Coated with β-Cyclodextrin Based Host-Guest Species through Resonant Energy Transfer from the Guests. RSC Adv 4(55): 28886-28892.
- Hao M, Sun G, Zuo M, Xu Z, Chen Y, et al. (2020) A Supramolecular Artificial Light‐Harvesting System with Two‐Step Sequential Energy Transfer for Photochemical Catalysis. Angew Chem 59(25): 10095-10100.
- Xun Z, Yu T, Zeng Y, Chen J, Zhang X, et al. (2015) Artificial Photosynthesis Dendrimers Integrating Light-Harvesting, Electron Delivery and Hydrogen Production. J Mater Chem A 3(24): 12965-12971.
- Wei X, Dong R, Wang D, Zhao T, Gao Y, et al. (2015) Supramolecular Fluorescent Nanoparticles Constructed via Multiple Non-Covalent Interactions for the Detection of Hydrogen Peroxide in Cancer Cells. Chem 21(32): 11427-11434.