Research Article
Creative Commons, CC-BY
Counteracting Action of Coutarea hexandra (Rubiaceae) Stem Bark Extract on the Systemic Toxicity Induced by Lachesis muta muta (Viperidae: Crotalinae) Venom in Rats
*Corresponding author: Floriano RS, Laboratory of Toxinology and Cardiovascular Research, Graduate Program in Health Sciences, University of Western São Paulo, Brazil, Email:rafael@unoeste.br
Received: September 13, 2021; Published: September 29, 2021
DOI: 10.34297/AJBSR.2021.14.001982
Abstract
Purpose: In this work, we investigated the protective action of Coutarea hexandra aqueous stem bark extract (Ch-E), associated with a commercial antivenom or as a stand-alone therapy, on the acute systemic envenomation induced by Lachesis muta muta in rats.
Methods: Male Wistar rats (300-350 g) were exposed to m. muta venom (1.5 mg/kg - i.m.) and then treated with a polyvalent antivenom (antivenom:venom ratio 1:3 ‘v/w’ - i.p.), Ch-E (100 mg/kg - i.p.) or combining both of these agents. After 120 min envenomation, animals were anesthetized in order to collect blood samples through intracardiac puncture and, subsequently, euthanized for collecting tissue samples; the hematological-biochemical and histopathological analyses were performed through conventional methods.
Results: Venom caused pronounced local oedema, necrosis formation and subcutaneous haemorrhage; Ch-E alone or combined with antivenom prevented the occurrence of subcutaneous haemorrhage. Ch-E administered alone or associated with antivenom effectively prevented the increase of serum creatine kinase (CK) and alanine aminotransferase (ALT) release; Ch-E reduced the release of the serum biomarker (CK-MB) for cardiotoxicity only in association with antivenom. Venom caused pronounced inflammatory responses associated with neutrophilia, eosinophilia and monocytosis; Ch-E alone also contributed significantly to reduce the venom-induced leukocytosis mainly due to its suppressive action on neutrophils. Ch-E prevented the venom-induced increase of reticulocytes either alone or in the presence of antivenom; however, no group had erythrocyte alterations. Ch-E produced effective protection against venom-induced hepatic and renal morphological alterations similarly to antivenom action.
Conclusion: Ch-E shows to be an important source of biomolecules with therapeutic potential to prevent efficiently a number of systemic disorders caused by m. muta venom in rats, e.g., myotoxicity, hepatotoxicity, inflammatory responses.
Keywords: Viperidae snake, L. m. muta venom, Systemic envenomation, C. hexandra extract, Antivenom property
Introduction
Genus Lachesis (Viperidae: Crotalinae) is represented by four terrestrial oviparous species, e.g., L. stenophrys, L. melanocephala, L. acrochorda and L. muta, being responsible by the second most frequent type of ophidian accident in Central and South America, after envenomations caused by Bothrops snakes [1,2]. In Brazil, there are two subspecies of Lachesis muta found in the Amazon river basin (L. m. muta) and Atlantic rainforest (L. m. rhombeata), [1,3] which occasionally cause severe human envenomation [4].
Envenomation by Lachesis spp. causes critical local [5-7] and systemic disorders characterized by haematological disturbances such as widespread bleeding, coagulopathy and pulmonary thrombosis [8-11], including renal failure [12], bradycardia and hypotension [8,13-16]. These venoms contain a wide variety of non-enzymatic proteins and peptides biologically active [17,18] including venom metalloproteases (SVMPs), serine proteases (SVSPs), phospholipases A2 (PLA2), C-type lectins and L-amino acid oxidase (LAAO), which are directly related to envenomation process by Lachesis spp. The earlier administration of a suitable polyvalent antivenom (anti-Bothrops/Lachesis serum) consists in the only therapeutic strategy available to attenuate or even preventing these clinical complications [19,20].
In recent years, some studies have demonstrated that plant extracts [21-23] and their isolated metabolites [24], including marine sponge extracts [25] might exhibit therapeutic properties to reduce some effects caused by Lachesis venoms. In addition, our group has also demonstrated that N-acetyl-L-cysteine (NAC), an antioxidant drug, contributes to minimize local and systemic disorders induced by L. m. muta venom in rats, e.g., myotoxicity, hepatotoxicity and inflammation, being a potential supporting therapeutic agent to antivenom therapy [26].
Coutarea hexandra, a Brazilian native plant found in the Atlantic rainforest [27], exhibits a range of therapeutic properties, e.g., suppressive activity on inflammatory responses, anti- nociceptive and anti-cancer actions [28,29], including potent antioxidant activity [30]. Based on these
positive aspects associated with the therapeutic functions of C. hexandra, and considering its ability in scavenging efficiently the stable radical 2,2-diphenyl-1-picrylhydrazyl [30], we aimed to investigate the counteracting action of C. hexandra aqueous stem bark extract (Ch-E) on the systemic effects induced by L. m. muta venom in rats. Ch-E was examined as a stand- alone therapy or in association with a polyvalent antivenom (anti-Bothrops/Lachesis serum), using haematological, biochemical, and histopathological approaches.
Materials and Methods
Reagents
Polyvalent antivenom (anti-Bothrops/Lachesis serum) was obtained from Instituto Butantan (São Paulo, SP, Brazil). Lachesis muta muta venom was provided by Center for Biological Studies and Research of the Pontifical Catholic University of Goiás (Goiânia, GO, Brazil) through Dr Nelson J. Silva Jr.; venom was obtained from one male adult snake from Altamira in the northern Brazilian state of Pará in the Brazilian Amazon (3°25′55′′S and 51°56′6′′W), lyophilized and stored at -20 °C until used. Coutarea hexandra bark extract was supplied by Tocantins Federal University (Palmas, TO, Brazil) through Dr Márcio G. Santos; the lyophilized extract was stored at -20 °C and resuspended in an aqueous solution before initiating the experimental protocols. The barks of C. hexandra were collected on June 2011 from a single tree georeferenced at S10º44’56.4” and W048º20’08.0” (elevation: 293 m), in Porto Nacional (TO, Brazil). Exsiccate no. 9708 was deposited in the Tocantins Herbarium located at Tocantins Federal University (Porto Nacional, TO, Brazil); routine procedure resulted in a 70% hydroalcoholic solution (v/v), 10% concentration (m/v), lyophilized and stored in an amber bottle.
Animals
Wistar rats (300-350 g; 2-3 months old) obtained from Central Bioterium of the University of Western São Paulo (UNOESTE, Presidente Prudente, SP, Brazil) were housed in plastic cages (2 animals/cage) with a wood-shaving substrate, at 23 ± 1 °C on a 12-h light/dark cycle with lights on at 6 a.m. The animals had free access to food and water. The experimental procedures were approved by an institutional Committee for Ethics in Animal Use (CEUA/UNOESTE, Protocol No. 6099) and were done according to the general ethical guidelines for animal use established by the Brazilian Society of Laboratory Animal Science (SBCAL) and Brazilian Federal Law No. 11.794 of October 8, 2008, in conjunction with The ARRIVE guidelines 2.0 [31].Experimental Design
The animals were transferred to the Experimental Bioterium of the University of Western São Paulo (UNOESTE, Presidente Prudente, SP, Brazil) and allowed to adapt for two weeks, under the same conditions as described above, before initiating the experimental procedures. The animals were then randomly distributed in six experimental groups (n = 6 per group) identified as: group G1 (control) - intramuscular (gastrocnemius muscle) injection of saline 0.9%; group G2 (Coutarea hexandra bark extract ‘Ch-E’) - intraperitoneal injection of Ch-E at a dose of 100 mg/kg; group G3 (L. m. muta venom) - intramuscular (gastrocnemius muscle) injection of venom at a dose of 1.5 mg/kg; group G4 (L. m . muta venom + antivenom) - intramuscular (gastrocnemius muscle) injection of venom ‘1.5 mg/kg’ followed by an intraperitoneal injection of antivenom at an antivenom:venom ratio of 1:3 (v/w); group G5 (L. m. muta venom + Ch-E) - intramuscular (gastrocnemius muscle) injection of venom ‘1.5 mg/kg’ followed by an intraperitoneal injection of Ch-E ‘100 mg/kg’; group G6 (L. m. muta venom + E-C.h. + antivenom) - intramuscular (gastrocnemius muscle) injection of venom ‘1.5 mg/kg’ followed by an intraperitoneal injection of Ch-E ‘100 mg/kg’ and antivenom ‘1:3 (v/w)’.
After 120 min, the animals were subsequently anesthetized by a non-lethal dose of thiopental (1.8 mg/kg, i.p.) and subjected to intracardiac puncture. Some blood samples were collected in vacuum EDTA tubes for hematological analysis and other ones in vacuum tubes without anticoagulant (BD Vacutainer®) for biochemical analysis; the animals were afterwards euthanized by an overdose of thiopental and subjected to dissection of gastrocnemius muscle (right ‘local of venom-injection’ and left ‘contralateral’ hind limbs), kidney, liver, lung and heart samples for histopathological analysis. To induce acute toxicity in rats, the dose of 1.5 mg of venom/kg was chosen based on previous investigations [26] and confirmed in pilot experiments in order to produce pronounced local and systemic alterations in at least 120 min post envenomation. The ability of anti-Bothrops/Lachesis serum produced by Instituto Butantan (IB) (São Paulo, SP, Brazil) to neutralize the L. m. muta venom-induced local and systemic effects in rats was assessed by administrating antivenom via intraperitoneal at an antivenom:venom ratio of 1:3 (v/w) immediately after exposing the animals to an intramuscular injection of venom (1.5 mg/kg); this antivenom:venom ratio was based on the manufacturer’s stated neutralizing capacity for the antivenom (1 ml of antivenom neutralizes 3 mg of L. muta venom). The effective dose (100 mg/kg) of Coutarea hexandra bark extract were determined in pilot experiments through serum CK measurements; there was no evidence for systemic toxicity produced by Ch-E alone (Figure. 1) summarizes the experimental design described in this section.
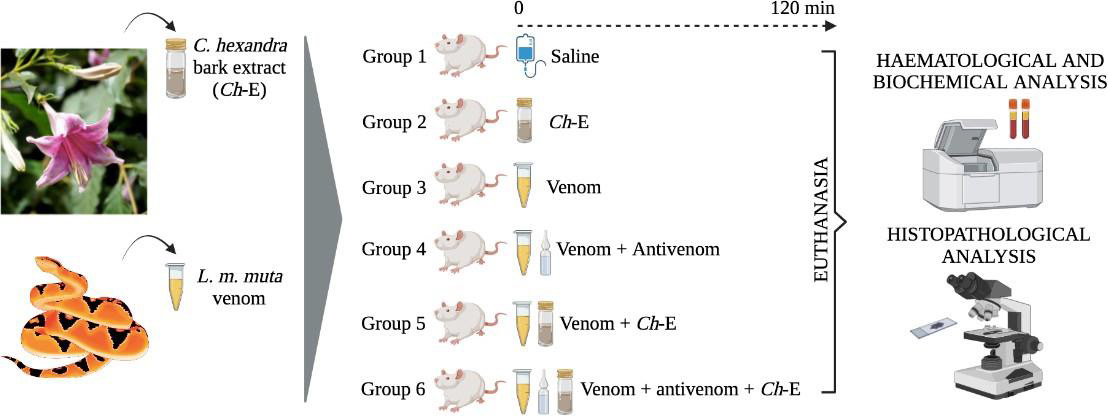
Figure 1: Experimental design for this investigation. In groups G4, G5 and G6, “+” indicates the combined action of venom vs. antivenom, venom vs. Ch-E or venom vs. antivenom and Ch-E, respectively
Haematological Analysis
Total leukocyte count was determined using the POCH-100 iV DIFF haematology analyser (Sysmex do Brasil Indústria e Comércio Ltda., São José dos Pinhais, PR, Brazil); for differential counting of leukocytes, stained blood smears were prepared using a Panótico® rapid staining (Laborclin Produtos para Laboratórios Ltda., Pinhais, PR, Brazil) and then analysed under an E-200 Nikon light microscope (Nikon Inc., Tokyo, Japan) at 100x magnification. The relative values for reticulocytes were determined as a percentage of total red blood cells [32,33]. The concentration of fibrinogen was determined by calorimetric technique using a refractometer ATCITREF- 200 (Instrutemp Instrumentos de Medição Ltda., São Paulo, SP, Brazil) and the results were expressed as mg/dL.
Serum Biochemical Analysis
The serum biomarkers for systemic myotoxic, cardiotoxic, hepatotoxic and nephrotoxic activities were determined using Cobas C111 commercial kits (Roche Holding AG, Basel, Switzerland) for creatine kinase ‘CK’ (code 07442017-190), creatine kinase myocardial band ‘CK-MB’ (code 05401763- 190), alanine aminotransferase ‘ALT’ (code 04718569-190) and creatinine ‘Cr’ (code 05401755-190), respectively. The assays were performed using a Cobas C111 analyser (Roche Holding AG, Basel, Switzerland).
Histopathological Analysis
After collecting the blood samples, the animals were subsequently euthanized by an overdose of thiopental and subjected to dissection in order to collect tissue samples from the gastrocnemius muscle, heart, lungs, liver and kidney. The samples were immediately fixed in 10% formaldehyde overnight and then washed for 30 min in 0.1 M phosphate-buffered saline and 30 min in distilled water prior to storage in 70% ethanol overnight. The samples were dehydrated in graded ethanol (80%, 95% and 100%), cleared in xylene (1:1 ethanol:xylene, 1:1 xylene:paraffin) and finally embedded in paraplast. Three sets of 2-5 sections (5 μm thick) per sample, separated from each other by 25 μm, were cut and mounted on plain glass slides for hematoxylin-eosin (HE) staining. Blind slide reading was carried out through a Leica ICC50HD camera coupled to a Leica DM750 light microscope (Leica Microsystems, Wetzlar, Germany) and the images were then captured and analyzed qualitatively using a LAS 4.2 software (Leica Microsystems, Wetzlar, Germany). The morphological changes and frequency of lesions were compared among the treatments based on a lesional score, as essentially described elsewhere [34,35].
Statistical Analysis
The results were expressed as the mean ± SEM and statistical comparisons were performed with Student’s t-test or oneway ANOVA followed by the Tukey test, with p < 0.05 indicated significance. In histopathological analysis, Dunn’s test was applied to evaluate the lesional score. All data were analyzed using Microcal Origin 8 SR4 v. 8.0951 (Microcal Software Inc., Northampton, MA, USA) and GraphPad Prism 4 v. 4.03 (GraphPad Software Inc., La Jolla, CA, USA) software.
Results and Discussion
Envenomations by Lachesis snakes are characterized by causing severe local damage and systemic disorders which commonly result in deaths, mostly associated with their potent cardiovascular action [8,13,15]. In this work, rats exposed to L. m. muta venom (1.5 mg/kg, i.m.) (G3) had intense local oedema, subcutaneous haemorrhage and necrosis formation (Table 1), accompanied by a number of systemic disorders such as myotoxicity, cardiotoxicity, nephrotoxicity, and hepatotoxicity as evidenced by significant increase in serum creatine kinase (CK), CK-MB (myocardial band), creatinine (Cr), and alanine aminotransferase (ALT) release, respectively, after 120 min envenomation (p < 0.05 compared to G1 ‘control’ for CK, CK-MB and ALT, n = 6) (Figure 2); such alterations were reflected by significant morphological damages (Figure 5). Venom also induced intense inflammatory response characterized by increase of total leukocytes which was reflected by increase of neutrophils, eosinophils, and monocytes after 120 min envenomation (p < 0.05 compared to G1 ‘control’ for all these parameters, n = 6) (Figure 3). The inflammatory activity induced by L. m. muta venom was also evidenced by increase in level of serum fibrinogen, while there was also an increase of reticulocytes most likely due to occurrence of subcutaneous haemorrhage (p <0.05 compared to G1 ‘control’ for both of these parameters, n = 6) (Figure 4), but without affecting the erythrocyte count [in mm3: 8.3 ± 0.3 (G1), 7.8 ± 0.3 (G2), 9.3 ± 0.2 (G3), 8.6 ± 0.2 (G4), 8.2 ± 0.2 (G5) and 8.3 ± 0.3 (G6), n = 6]. Such systemic alterations characterize the physiopathological profile produced by Lachesis venoms, as previously reported in studies with animal experimentation models [8,13,15,26] and in a human case report [36].
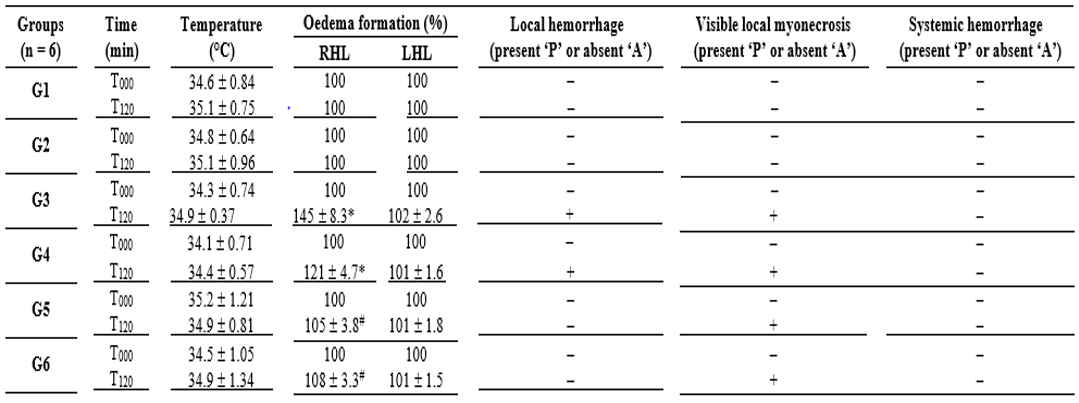
Table 1: Clinical aspects monitored during envenomation by Lachesis muta muta in rats treated with polyvalent antivenom and C. hexandra extract.
In Brazil, Lachesis snakebites are treated with polyvalent antivenoms consisted of immunoglobulins raised in hyperimmunized horses through a pool of venoms obtained from five Bothrops snake’s species (B. jararaca, B. alternatus, B. jararacussu, B. moojeni and B. neuwiedi) and Lachesis muta, including a second type of antivenom consisted of F(ab’)2 fragments from digested immunoglobulins raised in hyperimmunized horses using B. jararaca and L. muta venoms [26]. In group G4, the polyvalent antivenom therapy successfully prevented the venom-induced systemic myotoxicity, as indicated by increase of serum CK release, and acute hepatotoxicity by measuring serum ALT release (p < 0.05 compared to G3 ‘venom’ for both these biomarkers, n = 6) (Figure 2), and avoided the increase of reticulocytes release (p < 0.05 compared to G3 ‘venom’, n = 6) (Figure 4); however, antivenom only partially prevented the venom-induced cardiotoxicity, as measured through serum CK-MB release (Figure 2) and promoted limited protection against the increase in leukocytes involved in the inflammatory responses, e.g., neutrophils, eosinophils, and monocytes (Figure 3), including a mild protection against the increase of fibrinogen (Figure 4). In addition, antivenom showed to be efficient to decrease the venom-induced local myonecrosis, including renal and hepatic morphological damages (Figure 5).
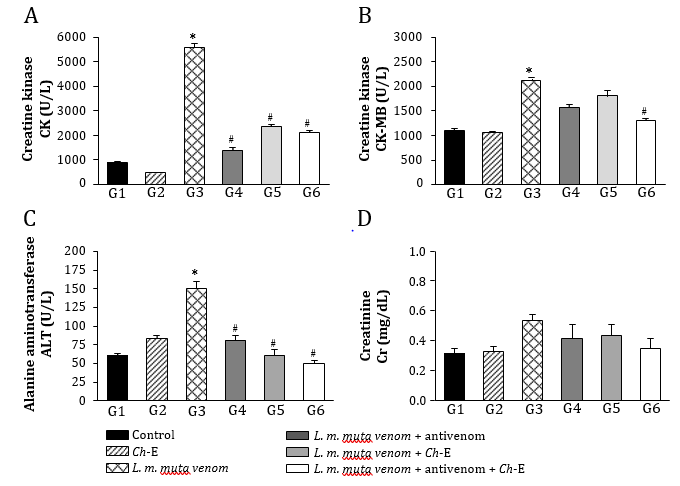
Figure 2: Analysis of blood-derived plasma protein biomarkers for systemic toxicity induced by L. m. muta venom in rats and treatment with antivenom and Ch-E. (A) Venom produced pronounced myotoxicity characterized by the increase of creatine kinase (CK) release, which was prevented by antivenom and Ch-E or by combination of both agents. (B) Venom-induced cardiotoxicity as assessed through CK-MB ‘myocardial band’ release into the blood was prevented only associating both of agents. (C) Venom caused hepatotoxicity characterized by increase of alanine aminotransferase (ALT) release, with all the treatments preventing the release of this biomarker. (D) There was no evidence for nephrotoxicity as assessed through creatinine release into the blood. The columns are the mean ± SEM (n = 6). *p < 0.05 compared to control (saline) group ‘G1’ and #p < 0.05 compared to venom group ‘G3’.
These polyvalent antivenoms comprise the only therapeutic strategy to neutralize the effects caused by L. muta (L. m. muta and L. m. rhombeata) venoms, with a few studies reporting the potential action of other antivenom supporting therapeutic tools regarding envenomations by Lachesis [23-25]. Recently, our group has demonstrated the benefits of the treatment with N-acetyl-L-cysteine, a drug known for eliminating a wide variety of reactive oxygen species and increasing the intracellular level of glutathione, on the local and systemic effects induced by L. m. muta venom in rats [26]. Based on these findings, we examined an eventual protective action by Ch-E on the experimental envenomation by L. m. muta in rats considering its various therapeutic applications [37-39], especially its antioxidant property [30]. C. hexandra, also popularly known as quina, exhibits diuretic and antipyretic activities, being commonly used to prevent intermittent fever caused by malaria and other infectious diseases [38,39]. Other activities such as anti-inflammatory [28,37], analgesic and antiviral [28] have been identified in C. hexandra. Here, as similarly demonstrated by antivenom therapy, Ch-E administered alone (G5) efficiently prevented the venom-induced increase of serum CK and ALT release (Figure 2), reduced inflammatory responses by decreasing leukocytosis and neutrophilia (Figure 3), including a slight reduction of fibrinogen (Figure 4) after 120 min envenomation (p < 0.05 compared to G3 ‘venom’ for all these parameters, n = 6). Compared to antivenom (G4), Ch-E administered alone (G5) or in association with antivenom (G6) only produced higher protection on the venom-induced leukocytosis and neutrophilia (p < 0.05 compared to G4 ‘antivenom’ for both of these parameters, n = 6) after 120 min envenomation (Figure 3). Ch-E when administered in association with antivenom produced greater protection on the venom-induced local myonecrosis, cardiac and renal morphological damages (Figure 5). Taken together, these data suggest a potential synergic action of these agents on some aspects of the envenomation by L. m. muta.
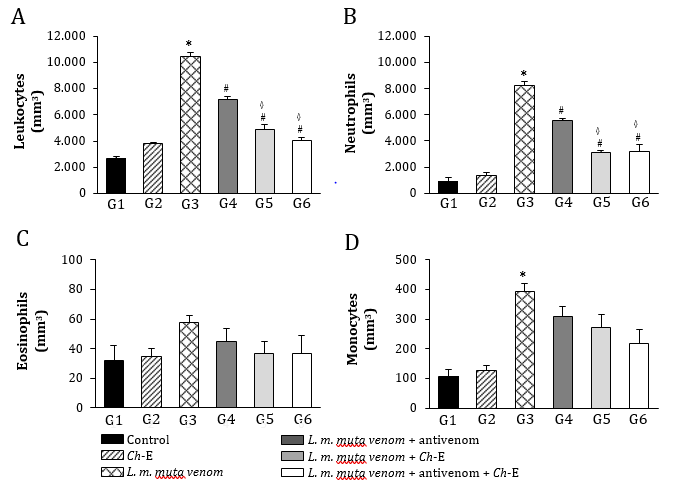
Figure 3: Leukocyte changes induced by L. m. muta venom in rats and treatment with antivenom and Ch-E. (A) Venom significantly increased the total leukocyte which was partially prevented by antivenom, with Ch-E alone or associated with antivenom producing higher protection. (B) Venom also promoted an increase of neutrophils, with Ch-E alone or associated with antivenom being again more effective than antivenom by itself. (C) There was no evidence for venom-induced eosinophilia. (D) Antivenom and Ch-E or their association failed to prevent the increase of monocytes induced by venom. The columns are the mean ± SEM (n = 6). *p < 0.05 compared to control (saline) group ‘G1’, #p < 0.05 compared to venom group ‘G3’ and ◊p < 0.05 compared to venom + antivenom group ‘G4’.
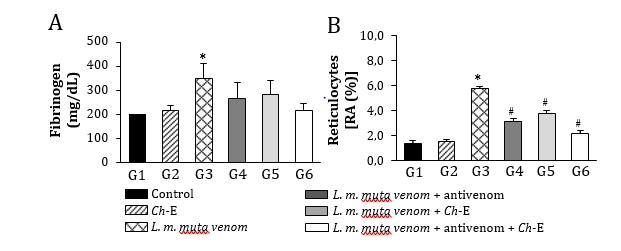
Figure 4: Fibrinogen and reticulocytes changes induced by L. m. muta venom in rats and treatment with antivenom and Ch-E. (A) Venom caused a discreet increase in fibrinogen level, which was unaffected for no treatment. (B) Venom altered the reticulocytes level, which was partially prevented by all the treatments. The columns are the mean ± SEM (n = 6). *p < 0.05 compared to control (saline) group ‘G1’ and #p < 0.05 compared to venom group ‘G3’.
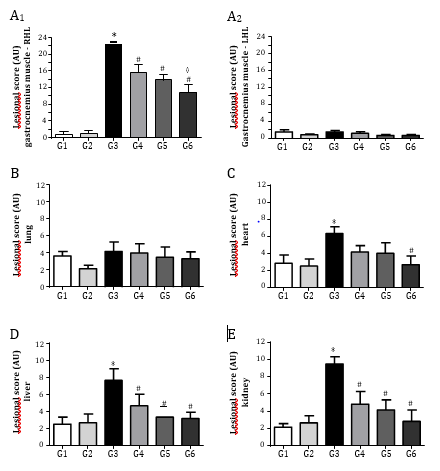
Figure 5: Morphological aspects of tissues samples dissected from rats exposed to L. m. muta venom and treated with Ch-E by itself or associated with antivenom. (A) Lesional score of right (local of venom injection – A1) and left (contralateral – A2) gastrocnemius muscles; venom caused noticeable local myonecrosis which was partially prevented by the single administration of antivenom and Ch-E, with their association being more effective on this parameter. (B–C) Venom did not cause relevant morphological changes in lung and heart tissue samples. (D) Venom produced moderate changes in lesional score of liver, with all the treatments effectively preventing the venom-induced hepatotoxicity. (E) Venom caused significant renal morphological changes, with all the treatments effectively preventing the venom-induced nephrotoxicity. *p < 0.05 compared to control (saline) group ‘G1’, #p < 0.05 compared to venom group ‘G3’ and ◊p < 0.05 compared to venom + antivenom group ‘G4’.
Extracts obtained from bark and leaves of C. hexandra exhibit a variety of phytochemical compounds such as vitamins, flavonoids, tannins, coumarins, steroids and alkaloids, which may be associated with several favourable physiological outcomes related to this plant [40]. However, C. hexandra still lacks to have its compounds isolated and systemically studied in order to better understand their mechanisms of action and consequences for human consumption
Conclusion
In conclusion, Ch-E shows to be an important source of biomolecules with therapeutic potential to prevent efficiently a number of systemic disorders caused by L. m. muta venom in rats, e.g., myotoxicity, hepatotoxicity, inflammatory responses, and renal and hepatic morphological damages when administered as a stand-alone therapy. In association with a commercial antivenom, Ch-E produced greater protection against the venom-induced leukocytosis and neutrophilia.
Acknowledgments
The authors thank Ana Maria S. S. Wehbe, Cleonice Trevisan and Gracielle V. Gonçalves for technical assistance. This work was funded by institutional resources from University of Western São Paulo (UNOESTE, Presidente Prudente, SP, Brazil). G.D. Pilon was supported by a studentship from Fundação de Amparo à Pesquisa do Estado de São Paulo (FAPESP, São Paulo, SP, Brazil, Grant No. 2019/21147-6).Conflict of Interest
The authors declare that they have no conflict of interest with this work.
References
- Diniz SR, Moraes JN, Rodrigues TM, Oliveira CS, Caldeira CAS (2020) A brief review on the natural history, venomics and the medical importance of bushmaster (Lachesis) pit viper snakes. Toxicon X 25(7):100053.
- Magalhães SFV, Peixoto HM, Moura N, Monteiro W, De Oliveira MRD (2018) Snakebite envenomation in the Brazilian Amazon: a descriptive study. Trans R Soc Trop Med Hyg 113(3): 143-151.
- Nogueira CC, Argôlo AJS, Arzamendia V, Azevedo JA, Barbo FE, et al. (2019) Atlas of Brazilian snakes: verified point-locality maps to mitigate the Wallacean shortfall in a megadiverse snake fauna. South American Journal of Herpetology 14(1): 1-274.
- O Sinan Sistema de Informação de Agravos de Notificação-SINAN.
- Damico DCS, Bueno LGF, Rodrigues SL, Marangoni S, Cruz HMA, et al. (2006) Functional characterization of a basic D49 phospholipase A2 (LmTX-I) from the venom of the snake Lachesis muta muta (bushmaster). Toxicon 47(7): 759-765.
- Damico DCS, Vassequi ST, Torres HFD, Nery DACC, Souza RCG, et al. (2012) LmrTX, a basic PLA2 (D49) purified from Lachesis muta rhombeata snake venom with enzymatic-related antithrombotic and anticoagulant activity. Toxicon 60(5): 773-781.
- Ferreira T, Camargo EA, Ribela MTCP, Damico DC, Marangoni S, et al. (2009) Inflammatory oedema induced by Lachesis muta muta (Surucucu) venom and LmTX-I in the rat paw and dorsal skin. Toxicon 53(1): 69-77.
- Dias L, Rodrigues MAP, Rennó AL, Stroka A, Inoue BR, et al. (2016) Hemodynamic responses to Lachesis muta (South American bushmaster) snake venom in anesthetized rats. Toxicon 123: 1-14.
- Estevão CMI, Diniz CR, Magalhães A, Markland FS, Sanchez EF (2000) Action of metalloproteinases mutalysin I and II on several components of the hemostatic and fibrinolytic systems. Thromb Res 99(4): 363-376.
- Rucavado A, Sánchez EF, Franceschi A, Magalhães A, Gutiérrez JM (1999) Characterization of the local tissue damage induced by LHFII, A metalloproteinase with weak hemorrhagic activity isolated from Lachesis muta muta snake venom. Toxicon 37(9): 1297-1312.
- Torres HFD, Werneck CC, Vicente CP, Vassequi ST, Nery DAC, et al. (2013) Rapid purification and procoagulant and platelet aggregating activities of rhombeobin: a thrombin- like/gyroxin-like enzyme from Lachesis muta rhombeata snake venom. BioMed Res Int. 903292.
- Damico DCS, Nascimento JM, Lomonte B, Ponce SLA, Joazeiro PP, et al. (2007) Cytotoxicity of Lachesis muta muta snake (bushmaster) venom and its purified basic phospholipase A2 (LmTX-I) in cultured cells. Toxicon 49(5): 678-692.
- Angel CKL, Guerrero VJA, De Carvalho EF, Lima SK, De Siqueira RJB, et al. (2020) Disorders on cardiovascular parameters in rats and in human blood cells caused by Lachesis acrochorda snake venom. Toxicon 184: 180-191.
- Diniz MR, Oliveira EB (1992) Purification and properties of a kininogenin from the venom of Lachesis muta (bushmaster). Toxicon 30(3): 247-258.
- Dias L, Rodrigues MAP, Inoue BR, Rodrigues RL, Rennó AL, et al. (2016) Pharmacological analysis of hemodynamic responses to Lachesis muta (South American bushmaster) snake venom in anesthetized rats. Toxicon 123: 25-44.
- Giovanni DSS, Aguiar AS, Gimenez AR, Novellino K, Soares MR (1997) Purification, properties, and N-terminal amino acid sequence of a kallikrein-like enzyme from the venom of Lachesis muta rhombeata (Bushmaster). J Protein Chem 16(8): 809-818.
- Pinheiro JEL, Boldrini FJ, De Campos ALMP, Santos FNA, Bendhack LM, et al. (2018) LmrBPP9: A synthetic bradykinin-potentiating peptide from Lachesis muta rhombeata venom that inhibits the angiotensin-converting enzyme activity in vitro and reduces the blood pressure of hypertensive rats. Peptides 102: 1-7.
- Pla D, Sanz L, Molina SP, Zorita V, Madrigal M, et al. (2013) Snake venomics of Lachesis muta rhombeata and genus-wide antivenomics assessment of the paraspecific immunoreactivity of two antivenoms evidence the high compositional and immunological conservation across Lachesis. J Proteomics 89: 112-123.
- Madrigal M, Pla D, Sanz L, Barboza E, Arroyo PC, et al. (2017) Cross-reactivity, antivenomics, and neutralization of toxic activities of Lachesis venoms by polyspecific and monospecific antivenoms. PLoS Neglected Trop Dis 11(8): e0005793.
- Solano G, Gómez A, Corrales G, Chacón D, Estrada R, et al. (2018) Contributions of the snake venoms of Bothrops asper, Crotalus simus and Lachesis stenophrys to the paraspecificity of the Central American polyspecific antivenom (PoliVal-ICP). Toxicon 144: 1-6.
- Oliveira DEC, Fernandes CP, Sanchez EF, Rocha L, Fuly AL (2014) Inhibitory effect of plant Manilkara subsericea against biological activities of Lachesis muta snake venom. Biomed Res Int 408068.
- Oliveira DEC, Cruz RAS, Amorim NM, Santos MG, Pereira LCS, et al. (2016) Protective effect of the plant extracts of Erythroxylum sp. against toxic effects induced by the venom of Lachesis muta snake. Molecules 21(10): 1350.
- Marques TR, Braga MA, Cesar PHS, Marcussi S, Correa AD (2019) Jabuticaba (Plinia jaboticaba) skin extracts as inhibitors of phospholipases A2 and proteases. An Acad Bras Ciências 91(2): e20180248.
- De Oliveira EC, Junior LCSP, Sanchez EO, Fuly AL (2020) Gallic and tannic acids potential against Lachesis muta venom toxic activities. Toxicon 177(Suppl 1): S29.
- Faioli CN, Domingos TF, De Oliveira EC, Sanchez EF, Ribeiro S, et al. (2013) Appraisal of antiophidic potential of marine sponges against Bothrops jararaca and Lachesis muta venom. Toxins 5(10): 1799-1813.
- Leão TAG, Pires CV, Ribelato AC, Zerbinatti MC, Santarém CL, et al. (2021) Protective action of N-acetyl-L-cysteine associated with a polyvalent antivenom on the envenomation induced by Lachesis muta muta (South American bushmaster) in rats. Toxicon 198: 36-47.
- Guia de Árvores da Mata Atlântica - GAMA. Coutarea hexandra (Jacq.) K.Schum.
- Lucena JEX, Bispo MD, Nunes RS, Cavalcanti SCH, Teixeira SF, et al. (2006) Efeito antinociceptivo e antiinflamatório do extrato aquoso da entrecasca de Coutarea hexandra Schum. (Rubiaceae). Rev Bras Farmacogn 16(1): 67-72.
- Olmedo D, Rodríguez N, Vásquez Y, Solís PN, López PJL, et al. (2007) A new coumarin from the fruits of Coutarea hexandra. Nat Prod Res 21(7): 625-631.
- Nunes LG, Gontijo DC, Souza CJA, Fietto LG, Carvalho AF, et al. (2012) The mutagenic, DNA-damaging and antioxidative properties of bark and leaf extracts from Coutarea hexandra (Jacq.) K. Schum. Environ Toxicol Pharmacol 33(2): 297-303.
- Percie SN, Ahluwalia A, Alam S, Avey MT, Baker M, et al. (2020) Reporting animal research: Explanation and elaboration for the ARRIVE guidelines 2.0. PLoS Biol 18(7): e3000411.
- Bessman JD 1990 Reticulocytes In: Walker HK, Hall WD, Hurst JW, editors. Clinical Methods: The History, Physical, and Laboratory Examinations. 3rd Boston, MA: Butterworths.
- Brandow AM 2018 Pallor and Anemia In: Kliegman RM, Lye PS, Bordini B, Toth H, Basel D, editors. Nelson Pediatric Symptom-Based Diagnosis. 20th Philadelphia, PA: Elsevier.
- Gerez JR, Pinton P, Callu P, Grosjean F, Oswald I, et al. (2015) Deoxynivalenol alone or in combination with nivalenol and zearalenone induce systemic histological changes in pigs. Exp Toxicol Pathol 67(2): 89-98.
- Grenier B, Loureiro BAP, Lucioli J, Pacheco GD, Cossalter AM, et al. (2011) Individual and combined effects of subclinical doses of deoxynivalenol and fumonisins in piglets. Mol Nutr Food Res 55(5): 761-771.
- Jorge MT, Sano MIS, Tomy SG, Castro SCB, Ferrari RA, et al. (1997) Snake bite by the bushmaster (Lachesis muta) in Brazil: case report and review of the literature. Toxicon 35(4): 545-554.
- Falcão HS, Lima IO, Santos VL, Dantas HF, Diniz MFFM, et al. (2005) Review of the plants with anti-inflammatory activity studied in Brazil. Rev Bras Farmacogn 15(4): 381-391.
- Lorenzi H (2002) Brazilian trees-manual identification and cultivation of woody plants native to Brazil [dissertation]. Nova Odessa (SP): Instituto Plantarum.
- Lorenzi H, Matos FJA (2002) Medicinal plants in Brazil: native and exotic cultivated [dissertation]. Nova Odessa (SP): Instituto Plantarum.
- Carvalho IMM (2009) Evaluation of antihepatotoxic activity of two plant species popularly known as "quina": Strychnos pseudoquina A. St. Hil. and Coutarea hexandra (Jacq.) K. Schum [dissertation]. Viçosa (MG): Universidade Federal de Viç