Research Article
Creative Commons, CC-BY
Analysis of the Mice Activity Profile in the Model of Spontaneous Aggression (MSA)
*Corresponding author: Viviane M S Fragoso, Laboratory of Innovations in Therapies, Education and Bioproducts, Oswaldo Cruz Foundation, Rio de Janeiro, RJ, Brazil.
Received: January 23, 2022; Published: February 25, 2022
DOI: 10.34297/AJBSR.2022.15.002136
Abstract
Stress is a physiological reaction that results from physical or psychological stimuli on the individual. The objective of this study was to investigate whether there is a connection between the hyperactivity profile before exposure to social stress and the appearance of highly aggressive behavior in the mice submitted at the Model of Spontaneous Aggression (MSA). The MSA used as the analysis of the activity profile of each individual the tail suspension test (TST). During the MSA, we observed different categories of behavior in response to social stress: aggressive (AgR), attacked (AgD), and harmonic. When verifying the influence of the activity profile observed in TST on aggressive behavior, we were seen a prevalence of the median mobility profile among the AgR animals, while the majority of animals with a hypoactive profile were categorized as AgD. Therefore, it was observed that there is no specific initial profile that indicates a tendency to become aggressive.
Keywords: MSA; Social Stress; Aggressiveness; Behavior
Introduction
According to the Ministry of Health, stress can be considered a natural reaction of the organism to situations of danger or threat caused by a psychological, environmental, or physiological stimulus, putting the individual on alert, generating physical or emotional changes (Ministry of Health, 2015). This physiological reaction is necessary to reestablish the organism’s homeostasis through the development of an effective adaptive response to stress. However, when an individual’s response to stress is seen as a threat to life or requires the individual’s body to develop an adaptive response to stress beyond that offered by the body, stress endangers the individual’s well-being (SENAP, 2019). Currently, stress can be divided into two types, depending on the time and intensity of the person’s exposure to the stressor (Antunes, 2019).
Stress affects the world population, mainly people who live in major cities, and it is the main cause of human social conflicts, for example, stress from socioeconomic status or work. Acute stress exposure can result in physical, biological, and mental damage, which affect multiple domains of the individual’s life. Chronic stress can result in changes in brain functions, changes in the neuroendocrine system, reductions in cellular differentiation and proliferation, and reductions in the survival of newly developed neurons produced in the region of the hippocampus (De Miguel et al., 2018; Stanisavljevic et al., 2019). The consequences of these social events of stress can be very harmful to health, especially the psychological suffering after exposure to the stressor; stress is a common risk factor for the development of many psychological disorders, such as anxiety, post-traumatic stress disorder, depression, and panic syndrome (McCall et al., 2015; Li et al., 2017; Antunes, 2019). These disorders are becoming more common, and aggressive behavior can present as one of the symptoms in humans.
According to the definition of the World Health Organization, aggression is considered the act(s) of an individual that harms or injures another/others of his kind (WHO 2017). There are different types of aggression, including impulsive aggression. This type of aggression is defined as unplanned aggressive behavior arising from a stimulus perceived by the individual. There is a strong emotional contribution, a reduction in the control of impulses, and a decrease in the perception of the intensity of the hostile act, leading to an exacerbation of aggression (de Almeida et al., 2015). This type of behavior can lead to consequences in society (de Vos et al., 2016), and as recently as 2015, in Brazil, the mortality rate due to aggression was 110.6 males and 7.0 females per 100 thousand inhabitants (Ministry of Health, 2017). This aggression resulting in death can be considered an exacerbated, pathological aggression, and some psychologists consider it violent (Yang et al., 2015; Cassiello-Robbins et al., 2015; Diliberto et al., 2016; Miles et al., 2016).
Violence is defined as the use of force (or power) against oneself, against another person, or against a group, characterized by the possibility of resulting in physical injury, psychological damage, and death. It promotes impairment in physical or emotional development and behavioral disorders in adulthood (WHO, 2017). Between 2005 and 2015, in Brazil, violence was responsible for an increase of approximately 10% in the homicide rate, and in 2015, there were 59,080 homicides (Cerqueira et al., 2018).
The study of violence between humans is extremely complex and involves biological, psychosocial, anthropological, and cultural factors (Campos et al., 2017). In mice, violence is studied through the analysis of aggressive behavior (Blanchard et al., 1988). Genetic changes in molecules that make up the HPA axis can also regulate their activation, resulting in the development, or not, of an effective adaptive response to stress. In a study by Gururajan et al. (2019), mice considered susceptible to social stress showed a significant increase in the expression of messenger RNA (mRNA) for corticotrophin-releasing factor (CRF) in the prefrontal cortex region but no change in mRNA expression for corticotropinreleasing factor receptor 1 (CRFR1) in any of the brain areas analyzed. However, they observed that mice considered resilient showed a significant increase in CRFR1 mRNA expression in the hippocampal region and a significant reduction in CRF mRNA expression in the prefrontal cortex region (Gururajan et al., 2019).
Cellular components, such as mitochondria, can also suffer genetic changes and influence the development of aggressive behavior in individuals subjected to stress. A study carried out in mice subjected to stress showed that animals exposed to the stressor demonstrated a significant increase in the expression of genes encoded by mitochondrial DNA (mtDNA) in the prefrontal cortex region for all complexes present in oxidative phosphorylation, except complex II. One of the explanations suggested by the author was that this increase in the expression of genes encoded by mtDNA would be a cellular response to reduce the effects of stress caused in the cell. However, genetic changes or mitochondrial functional capacity can cause a change in the physiological response to stress (Weger et al., 2020).
Thus, it is necessary to develop experimental models that allow investigation of the genesis of aggressiveness to understand and prevent aggressive behavior that arises from a situation of social stress and consequently minimize the number of violent events in society. During the observation of the behavior of adult male Swiss Webster mice in housing facilities (under space restriction of cages), we observed highly aggressive individuals in certain groups, and interestingly, in other groups under the same environmental conditions, there was harmonious coexistence between individuals (Batista et al., 2012).
These facts stimulated us to develop, in 2012, an innovative behavioral analysis technique called the Model of Spontaneous Aggressiveness (MSA). The model was created to investigate the evolution and causes of aggressive behavior in mice of the Swiss Webster lineage in our housing facilities. The MSA was validated (Fragoso et al., 2016) and used in others our group’s works (Mendonça et al., 2019; Felippe et al., 2021). It is based on the grouping of these animals around weaning and on regrouping in adulthood, and the regrouping is a stressor that may cause aggressive behavior (Fragoso et al., 2016). One of the behavioral tests in MSA is the tail suspension test (TST). The TST as a selection method for regrouping (adult males’ new groups), and it is used to identify the activity profile of each animal. Through this methodology, we are investigating the mechanisms that may be involved in the regulation and genesis of exacerbated aggressive behavior in individuals under social stress. Therefore, we decided to investigate in this study whether the hyperactive profile, observed in some animals during the TST, can influence the emergence of aggressive behavior that some MSA mice show after exposure to social stress.
Methods
Animals
Male conventional Swiss Webster mice (n=116) from the Institute of Science and Technology in Biomodels (ICTB/ FIOCRUZ) were used. The animals were received and adapted to environmental conditions for five consecutive days. For each small polycarbonate cage, four individuals were housed. The mice were kept in the LBC/LITEB Experimental Animal Facility (Oswaldo Cruz Institute) under the following conditions: humidity (40 to 50%), temperature (20 to 22 °C), air exchange (estimated at 25 changes/ hour) and photoperiod (12 h day/12 h night). The floor of the cage was covered with wood shavings that were changed twice a week. In addition, animals were offered filtered water and autoclaved feed ad libitum. No environmental enrichment was offered. All procedures described in this project are in accordance with Brazilian Laws and ethical principles approved by License number CEUA/IOC-032/2019-A1.
Noninvasive Parameters
Model of Spontaneous Aggression (MSA): At 3 weeks old (wko), the animals were divided into 6 groups (A1 to A6), with 5 animals each, individually identified (C1 to C5). At 4, 6 and 8 wko, we performed the tail suspension test (TST), as described by Steru et al. (1985), ethogram and pattern of aggressive behavior (PAB). At 10 wko, we regrouped these animals into 5 groups (R1 to R5) containing 4 animals each, using the following criteria for the composition of the group: 1 hyperactive individual (Hyper), 1 hypoactive individual (Hypo) and 2 medium animals (Med). A cage containing 5 animals not regrouped (NR) became our negative control for social stress. At the 12th,14th and 16th weeks of life, the ethogram and pattern of aggressive behavior test were repeated, and the animals were categorized into harmonics (Har), animals with reduced aggression and discrete or no bites/lesions on the body; defeated animals (AgD), animals that present moderate lesions on the body resulting from fights and aggressions; and aggressors (AgR), individuals that show high aggressiveness in relation to other individuals in the group, facilitating the reproducibility of aggressive behavior among animals (Figure 1). All MSA were repeated four times. The animal study was reviewed and approved by the Animal Use Ethics Committee of the Oswaldo Cruz Institute (CEUA/IOC) under license number 032/2019-A1.
Three-week-old male Swiss Webster mice were grouped into five groups (n = 5 in each group), and behavioral tests, such as the tail suspension test (TST), ethogram and pattern of aggressive behavior (PAB), were performed at the 4th, 6th, and 8th weeks of life. At the 10th week of life, through mobility in the TST, they were classified as hyperactive (High), hypoactive (Low) and medium mobility (Med) and selected for regrouping. Some of the animals were regrouped into five groups with one high animal, one low animal and three Med animals in each group. Regrouping represents a situation of social stress for animals. A group of 5 animals was not regrouped (NR). They served as the social stress negative control. In the 12th, 14th and 16th weeks of life, we performed aggressive ethograms and PAB. At the 16th week of life, the regrouped animals were categorized as harmonic (Har), defeat or attacked (AgD) and highly aggressive (AgR). All MSA were repeated four times.
Tail Suspension Test (TST)
To select the new groups, animals were suspended by the final third of the tail at the top of the apparatus, and the amount of the five-minute test time the animal maintained a posture of mobility/immobility to this situation was quantified (Steru et al., 1985). Three categories were statistically defined related to the animal’s physical reaction: a) low activity (hypoactive), 104 to 150 seconds of immobility; b) median activity (medians), between 51 and 103 seconds of immobility; and c) high activity (hyperactive), 0 to 50 seconds of immobility. This test was used to standardize the regrouping so that each cage had one hyperactive, one hypoactive, and two medium animals.
Pattern of Aggressive Behavior (PAB)
As described by Fragoso et al. (2016), we use the ethogram as a tool for observing animal behavior in the grouping and regrouping phases. The ethogram is based on continuous filming (top view) using a Canon PowerShot SX20 IS® camera (Lake Success, New York, USA) of each group of animals for 30 minutes. This made it possible to evaluate the degree of aggression characterized and assessed by quantifying the number of attacks and measuring the extent of the lesion with a digital caliper (Digimess, China), making it possible to calculate the area (cm²) injured by the attacks. PCA was performed through the individual physical inspection of all animals, enabling the quantification of aggression according to predetermined scores: (0) mice with no bites or injuries on the body; (1) mildly aggressive nonsexual events and discrete bite signs on the back or tail; (2) aggressive events and moderate lesions on the tail, back and scrotum; and (3) severely aggressive events and marked lesions on the tail, back and urogenital region.
Analysis of Behavioral Profile
During the MSA grouping, the individual results of the mice were recorded in the TSTs performed at the 4th, 6th, and 8th weeks of life, and these animals were classified at the 10th week of life. In the regrouping, the individual results of the mice were also recorded for the characterization of the degree of aggression performed at the 12th and 14th weeks of life, and they were categorized at the 16th week of life. After the completion of the MSA, these notes were compiled in tables, and an analysis of the relationship of the profiles of the mice in the grouping and regrouping phases was carried out. The correlation between the individual classification of the mice at the 10th week of life and their categorization at the 16th week of life were also analyzed and compiled in graphs, where it was possible to evaluate the percentage of animals with high, low, and median mobility in each categorized group (Har, AgD and AgR) and the not regrouped group (NR).
Statistical Analysis
Data are presented as the mean ± SD or % values for each condition. The Wilson/Brown method (99% confidence intervals) was applied to analyze the percentage of the total fraction. Comparisons between groups were performed by nonparametric Kruskall-wallis followed by Dunn’s multiple comparisons test by using GraphPad Prism software version 8.0.1 for Windows (GraphPad Software, USA). Differences of p<0.05 were considered to be significant.
Results
Animal Categorization
Swiss Webster mice were submitted to MSA to investigate the degree of aggressive behavior under social stress. The animal’s susceptibility to a mild stress situation was measured by the mobility time in the TST. Three categories were determined through the use of statistical parameters: hyperactivity, hypoactivity and median activity. PAB was also evaluated. Our results showed that in the original mouse group, only one animal had a score of 1, and the rest had a score of 0 during the tests at 4, 6, and 8 wko. There were no aggressive events and no injuries in the groups (data not shown). In relation to the TST, i) 41% of the animals had median mobility, ii) 36% were hyperactive, and iii) 22% of the animals were hypoactive (Fig. 2A). In none of these groups was any degree of aggressiveness observed. After the adult male Swiss Webster mice were regrouped, the PAB analysis made it possible to categorize the animals according to the levels of aggressiveness under social stress: i) Harmonic mice (Har), individuals that showed no degree of aggressiveness, ii) Defeat or attacked individuals (AgD), animals that suffer attacks and bites and iii) Highly aggressive mice (AgR). The intensity of aggressive behavior can be described as follows: a) AgR animals attacked other animals on average (7.4 ± 5.0 attacks/30 min) (p ≤ 0.05) (Fig. 2B); b) consequently, the AgD animals had significant fight lesions with medium extension (5.6 ± 3.9 cm²) (p ≤ 0.05) in relation to the other categories that did not suffer aggression (Fig. 2C). The PAB showed that the subordinated animals (AgD) had a score significantly higher than 2.0 ± 0.7, in relation to the other categories (NR: 0.0 ± 0.0; Har: 0.2 ± 0.1; AgR: 0.0 ± 0.0) (Fig. 2D).
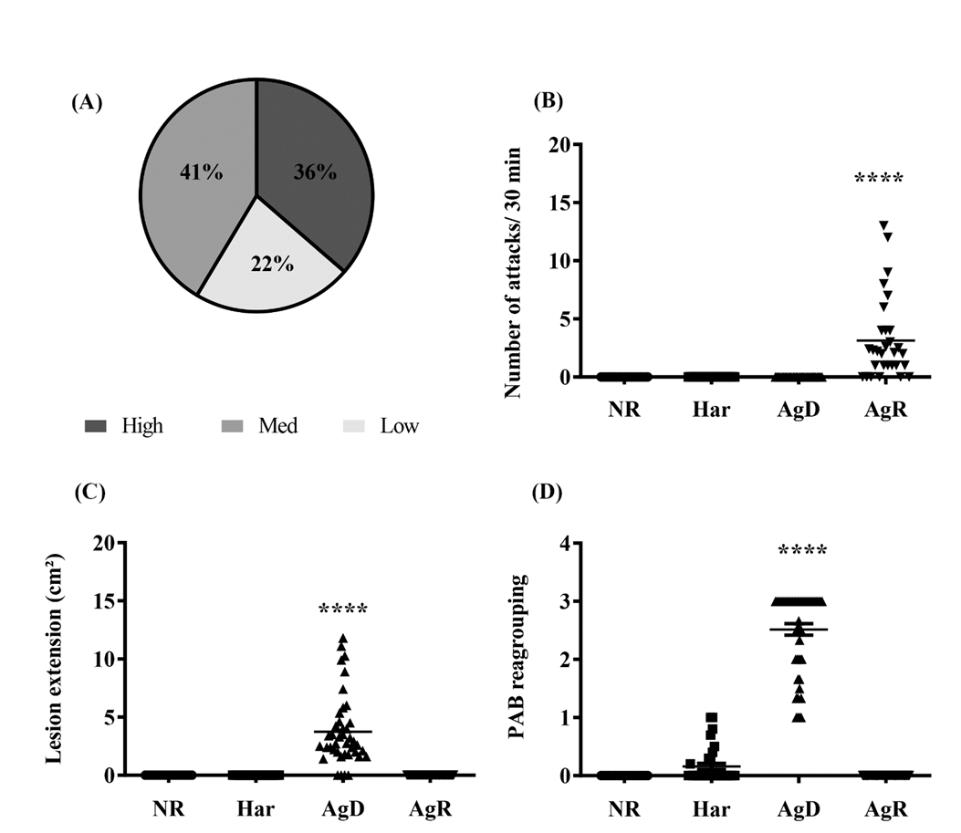
Figure 2: Evaluation of behavioral parameters in social stress. (A) Distribution animal’s according to the mobility profile obtained by TST for regrouping in the 10th week of life. In the grouping we obtained: Dark green: Hyperactive (36%); Green: Medium (41%); Light green: Hypoactive (22%); (B) Number of attacks/30 min according to the categorization of animals in NR (not regrouped), Har (harmonic), AgD (atacked) and AgR (aggressive); (C) Lesion extension (cm2) according to the behavioral categories of animals in NR, Har, AgD and AgR; (D) Evaluation of the pattern of aggressive behavior (PAB) after the regrouping. The values were expressed according to the individual results of the mice from four independent experiments. **** Corresponds to the statistical significance (p <0.0001) between AgR and the other categories and AgD and the other categories by the nonparametric Kruskal-Wallis and Dunn’s tests.
Does the TST profile show a direct correlation with aggressive behaviors?
In the present study, we sought to investigate whether the social stress caused by regrouping would be able to cause changes in the animal’s behavioral profiles observed in TST. In summary, we compared the mobility of animals in grouping and regrouping (see supporting information). Our results showed that 42.8% of AgR mice had medium mobility, 37.1% had hyperactivity, and 20% had a hypoactive profile (Fig. 3A); 35.1% of AgD mice showed hypoactivity, 35.1% had hyperactivity, and 29.7% had a medium mobility profile (Fig. 3B). A total of 41.6% of animals in the category of Har had medium mobility, 37.5% had hyperactivity and 20.8% had a hypoactive profile (Fig. 3C). In the NR group (without social stress), 60.0% had median mobility, 35.0% had a hyperactive profile, and 5.0% had a hypoactive profile (Fig. 3D).

Figure 3: Influence of the mobility profile in the categorization of animals submitted to MSA. After MSA, each animal’s activity profile in the tail suspension test and categorization after regrouping were analyzed. (A) Thirty-five animals were considered AgR, 42.86% with moderate mobility, 37.14% high mobility, and 20% low mobility. (B) Thirty-seven animals were considered AgD, 35.13% had a low mobility profile, 35.13% had high mobility, and 29.73% had medium mobility. (C) Twenty-four animals were considered Har, 41.67% with med mobility, 37.50% high mobility, and 20.83% low mobility. (D) Twenty animals remained in the not regrouped group (NR): 60% with moderate mobility, 35% with high mobility, and 5% with low mobility.
Discussion
In this study, we investigated whether exposure to social stress can influence the appearance of aggressive behavior in individuals that previously had a hyperactive mobility profile in TST.
Our results demonstrate that under social stress, the majority of animals considered aggressive (AgR) presented a medium mobility profile during grouping. Other interesting results were that most of the attacked animals (AgD) showed a hypoactive profile before regrouping and without social stress, and only 5% showed a hypoactive profile in the TST at 4, 6 and 8 wko.
Although some hyperactive individuals develop aggressive behavior, after the social stress of regrouping, most animals were divided among the other categories. That is, social stress is an environmental factor capable of causing behavioral changes in individuals exposed to it, without a prior profile for aggressiveness. In addition to the fact that stress can stimulate aggressive behavior in animals exposed to it, other factors can also indirectly interfere with the appearance of this aggressiveness in individuals after exposure to stress.
Genetic factors can influence the individual’s predisposition to aggression after this individual is exposed to stress. Genetic differences (such as polymorphism) in genes that code for the oxytocin receptor (OXTR) may influence the appearance of antisocial behavior in individuals after exposure to a stressor (Sicorello et al., 2020; Poore & Waldman, 2020). One of the functions of oxytocin, a hormone produced by the hypothalamus, is to develop attachment and empathy among people (Campos & Graveto 2010; Bonassi et al., 2020). In a study carried out with humans, it was observed that the population that had been subjected to stress and had a genetic polymorphism for OXTR developed antisocial behavior, even after being removed from the stressful situation (Sicorello et al., 2020).
Other hormones important for the physiological stress response, such as cortisol, can also exhibit genetic changes. Cortisol is a hormone released into the bloodstream through the activation of the hypothalamic-pituitary-adrenal axis (HPA axis). The activation of this axis is the main response to stress developed by the body to recover homeostasis. Some brain regions, including the hippocampus, modulate the activation or inhibition of the HPA axis through neural projections. The hippocampus projects to the paraventricular nucleus of the hypothalamus to inhibit the activation of the HPA axis.
The neuroendocrine response elicited by social stress is similar in humans and mice, and cortisol (in humans)/corticosterone (in mice) and dopamine are molecules that modulate aggressive impulsive responses. In mice subjected to MSA, our group observed that, in contrast to the subordinate and harmonic mice, aggressive mice were not able to increase their production of corticosterone in a situation of stress. This finding suggests that these aggressive mice have a deficiency in the ability to promote a good adaptive response to stress (Campos et al., 2017).
In addition, when analyzing the release of dopamine, serotonin, and norepinephrine in the regions of the amygdala, hippocampus, and prefrontal cortex using high-performance liquid chromatography (HPLC), it was observed that the amount of dopamine (mean value of all regions) was higher in AgR animals than in the other categories. In the prefrontal cortex region, the increase in dopamine levels was up to 85% higher in AgR than in harmonic mice. Additionally, there was an increase in the difference in the serotonin/dopamine relation in all regions of the animals, showing an imbalance between these neurotransmitters and an increase in dopamine, suggesting that dopamine is related to the emergence of exacerbated aggressiveness (Oliveira et al., 2014).
Given these results, our group investigated whether the use of dopamine 2 receptor (D2R) blockers could interfere with the aggressive behavior of animals that became highly aggressive in MSA. Using the first-generation antipsychotic haloperidol (which acts as a D2R antagonist) and the second-generation antipsychotic risperidone (which acts as an antagonist on D2R and the 5-HT1A and 5-HT2A serotonergic receptors), there was a significant decrease in the number of attacks promoted by animal aggressors, and the extent of the injury in the victims was approximately 63% with the administration of haloperidol and 95% with risperidone. Furthermore, after interruption of pharmacological treatment for 10 days, the aggressive behavior and the number of attacks increased by 26% with the interruption of haloperidol and 25% with risperidone. Thus, we suggest that the reduction in aggressive behavior was mainly due to the blockade of D2 receptors and that the dopaminergic system is involved in this process (Fragoso et al., 2016).
It is known that the increase in dopamine could interfere with mitochondrial oxidative phosphorylation (Czerniczyniec et al., 2007; Hankin et al., 2015), decreasing the activity of cytochrome c oxidase subunit IV (COX IV). Stress can cause intracellular changes, including in mitochondria (Tielbeek et al., 2018). Thus, our group investigated whether there was any relation between the susceptibility to stress, the appearance of highly aggressive behavior, and the adaptability of mitochondrial metabolism in the brains of mice subjected to MSA. High-resolution respirometry was used to observe a reduction of approximately 43% in the activity of cytochrome c oxidase (COX) in the frontal cortex of AgR animals compared to NR animals. By spectrophotometry, the enzymatic reduction was approximately 70% greater in the aggressive animals than in the control group (Mendonça et al., 2019).
Therefore, the activation of the dopaminergic system, possibly due to alterations in the expression of dopaminergic receptors 1 and 2 (D1R and D2R) and the elevation of the dopamine concentration, suggests that it promotes mitochondrial dysfunction, such as a decrease in the enzyme activity of Cytochrome C oxidase subunit IV (COX IV) in the mitochondria.
The main objective of our current study was to correlate animal mobility (when exposed to a mild stress situation by the TST) with the degree of aggression that animals exhibited on regrouping, that is, under social stress. Interestingly, we did not observe the correlation we expected. The population of individuals with high mobility (hyperactive) was not the population with exaggerated aggressiveness during social stress. However, the animals with less activity (hypoactive) were the animals that suffered the greatest number of aggressions (AgD) under social stress. Our conclusion will be based on this summary:
1) BfRcat: 60% medium; 35% high and 5% hypoactivity
Not submit to social stress and absence of aggression
2) HARcat: 41,6% medium; 37,5% high and 20,8% hypoactivity
Submit to social stress and absence of aggression
3) AgRcat: 42,8% medium; 37,1% and 20% hypoactivity
Submit to social stress and high aggressive
The first conclusion that these data demonstrate is that the regrouping of adult males is a strong factor of social stress in mice in a house facility. Modifies your activity profile by raising the percentage of the population of hypoactivity (depression-like) and hyperactivity (anxiety-like) in the new group. However, one question we could not elucidate. HAR and AgR groups have a similar mice activity profile in their population. Because some individuals have exacerbated aggression? Without being able to predict, behaviorally, which individual will be highly aggressive. Maybe there is a set of environmental, genetic, and physiological factors that interact with each other, forming a complex and extensive connection between them and resulting in more aggressive behavior under social stress. Therefore, this study demonstrated the importance of the experimental model to investigate aggressive behavior genesis under social stress, which we deem as immense and extremely complex.
Acknowledgment
The authors thank the Laboratory of Innovations in Therapies, Education and Bioproducts and the Laboratory of Cell Biology for technical support and discussions that helped in understanding the results obtained as well as the Graduate Program in Cell and Molecular Biology for making possible the development of this work. This work was financed in part by the Coordination for the Improvement of Higher Education Personnel - Brazil (CAPES) - Financial Code 001, the Laboratory of Innovations in Therapies, Education and Bioproducts, and the Oswaldo Cruz Foundation.
References
- Ministry of Health. Estresse. Biblioteca virtual em saúde.
- SENAP (2019) Caderno técnico de tratamento do transtorno de estresse pós-traumático – TEPT / Ministério da Justiça e Segurança Pública, Secretaria Nacional de Segurança Pública.
- Antunes J (2019) Estresse e doença. O que diz a evidência? Psicologia, saúde & doenças, 20(3): 590-603.
- Stanisavljevic A, Perić I, Gass P, Inta D, Lang UE, et al. (2019) Brain Sub/ Region-Specific Effects of Olanzapine on c-Fos Expression of Chronically Socially Isolated Rats. Neuroscience 396: 46-65.
- McCall JG, Al-Hasani R, Siuda ER, Hong DY, Norris AJ, et al. (2015) CRH Engagement of the Locus Coeruleus Noradrenergic System Mediates Stress-Induced Anxiety. Neuron 87(3): 605-620.
- Piao C, Deng X, Wang X, Yuan Y, Liu Z, et al. (2017) Altered function in medial prefrontal cortex and nucleus accumbens links to stress-induced behavioral inflexibility. Behavioural Brain Research 371: 16-26.
- World Health Organization. Violence [Internet]. Geneva: WHO 2017.
- de Almeida RMM, Cabral JCC, Narvaes R (2015) Behavioural, hormonal and neurobiological mechanisms of aggressive behaviour in human and nonhuman primates. Physiology & Behavior 143: 121-135.
- de Vos MEK, Bernstein DP, Vanstipelen S, de Vogel V, Lucker TPC, et al. (2014) Schema modes in criminal and violent behaviour of forensic cluster B PD patients: A retrospective and prospective study. Legal and Criminological Psychology 21(1): 56-76.
- Yang CR, Bai YY, Ruan CS, Zhou HF, Liu D, et al. (2015) Enhanced Aggressive Behaviour in a Mouse Model of Depression. Neurotoxicity Research 27(2): 129-142.
- Cassiello-Robbins C, Conklin LR, Anakwenze U, Gorman JM, Woods SW, Shear, et al (2015) The effects of aggression on symptom severity and treatment response in a trial of cognitive behavioral therapy for panic disorder. Comprehensive Psychiatry 60: 1-8.
- Diliberto RA, e Kearney CA (2016) Anxiety and oppositional behavior profiles among youth with selective mutism. Journal of Communication Disorders 59: 16-23.
- Miles SR, Menefee DS, Wanner J, Tharp AT, e Kent TA (2016) The Relationship Between Emotion Dysregulation and Impulsive Aggression in Veterans With Posttraumatic Stress Disorder Symptoms. Journal of Interpersonal Violence 31(10): 1795-1816.
- Cerqueira DRC, Lima RS, Bueno S, Neme C, Ferreira H, et al. (2018) Atlas da Violência 2018. IPEA.
- Campos JDS, De Araújo CFM, Hoppe LY, Fragoso VMS, e Oliveira GM (2017) Desenvolvimento de um Modelo Experimental para o Estudo da Violência através do Comportamento Agressivo de Camundongos da Linhagem Swiss Webster em Biotério. Revista- Sociedade Brasileira de Ciências em Animais de Laboratório 5: 52-63.
- Blanchard RJ, Hori K, Blanchard DC (1988) Social dominance and individual aggressiveness. Aggression Behavior 14(3): 195-203.
- Gururajan A, Van WM, Boehme M, Becker T, O'Connor R, et al. (2019) Resilience to Chronic Stress Is Associated with Specific Neurobiological, Neuroendocrine and Immune Responses. Brain, Behavior, and Immunity 80: 583-594.
- Weger M, Alpern D, Cherix A, Ghosal S, Grosse J, et al. (2020) Mitochondrial gene signature in the prefrontal cortex for differential susceptibility to chronic stress. Scientific Reports 10: 18308.
- Batista WS, Da Silva LCCP, Demarque KC, Oliveira FS, Acquarone M, et al. (2012) Estudo do comportamento agressivo de camundongos em biotério: Aplicação do Modelo Espontâneo de Agressividade (MEA). Revista- Sociedade Brasileira de Ciências em Animais de Laboratório 1(4): 322-335.
- Mendonça APM, Hoppe LY, Gaviraghi A, Araújo-Jorge TC, Oliveira GM, et al. (2019) Highly aggressive behavior induced by social stress is asociated to reduced to cytochrome c oxydase activity in mice brain cortex. Neurochemistry International 126: 210-217.
- Felippe RM, Oliveira GM, Barbosa RS, Esteves BD, Gonzaga BMS, et al. (2021) Experimental Social Stress: Dopaminergic Receptors, Oxidative Stress, and c-Fos Protein are involved in highly aggressive behavior. Frontiers in Cellular Neuroscience 15: 696834.
- Steru L, Chermat R, Thierry B, e Simon P (1985) The tail suspension test: a new method for screening antidepressants in mice. Psychopharmacology 85: 367-370.
- Sicorello M, Dieckmann L, Moser D, Lux V, Luhmann M, et al. (2020) Oxytocin and the stress buffering effect of social company: a genetic study in daily life. Social Cognitive and Affective Neuroscience 15(3): 293-301.
- Poore HE, Waldman ID (2020) The Association of Oxytocin Receptor Gene (OXTR) Polymorphisms Antisocial Behavior: A Meta-analysis. Behavior Genetics 50(3): 161-173.
- Campos DCF, Graveto JMGN (2010) Oxitocina e comportamento humano. Revista de Enfermagem Referência, Coimbra, v. serIII 1: 125-130.