Case Report
Creative Commons, CC-BY
Molecular Modeling, β-Hematin Interactions Evaluation Based on Antimalarial Compounds and Synthesis of Some Hydroxypyridinone Derivatives as New Scaffold
*Corresponding author: Neda Fayyazi, Department of Medicinal Chemistry, School of Pharmacy and Pharmaceutical Sciences, Isfahan, Iran. Email: fayyazi_neda@gmail.com.
Lotfollah Saghaei, Department of Medicinal Chemistry, School of Pharmacy and Pharmaceutical Sciences, Isfahan, Iran. Email: saghaei@pharm.mui.ac.ir.
Received: May 10, 2022; Published: June 02, 2022
DOI: 10.34297/AJBSR.2022.16.002231
Abstract
The principal mechanism in blood stage in malaria parasite is hematin crystallization which is the viable target of the antimalaria compounds. Molecular modeling, qualitative pharmacophore and docking evaluation of some reported and new derivatives such as acridinone were also performed to investigate binding interactions with the heme sheet. Moreover, a series of hydroxypyridinone analogues were designed and synthesized using the structure-based approach to develop new antimalaria compounds. A series has been synthesized and characterized by FT-IR, NMR spectroscopy and mass spectrometry. Our compounds with appropriate features were evaluated for β- hematin inhibitors compared to the chloroquine as an antimalarial compound. The structure-based model and biological activities have shown axial Fe-interactions and π-π stacking in synthesized compounds. The substitution of synthesized imines (5a-5c) and amines (6a-6c) compounds based on hydroxypyridinone evaluated with alteration lateral chains aromatic for inhibition of β-hematin formation according to the results of molecular docking. Hydrophobic and H-bond acceptor features of pharmacophore are essential to show the best biological activity that have helped to develop a pharmacophore model based on 2-ethylphenyl imino-substituted hydroxypyridinone. Also, these compounds were evaluated for their β-hematin inhibition activity.
Keywords: β-hematin, Molecular Interactions, Antimalarial, ADMET, Hydroxypyridinone
Introduction
For many years, malaria has posed serious threat to the health of the poorer nations and has inflicted massive costs in the affected regions [1]. Anopheles parasites associated with the genus Plasmodium is responsible for a wide range of cumbersome ailments around the world [2]. The risk of the malaria infection threatens approximately 40% of the world population with 300-660 million clinical experiences of Plasmodium falciparum malaria occurring each year [3]. During erythrocytes lifecycle, heme is released from hemoglobin as food source. Detoxification of heme is completed by oxidization and conversion to ferriprotoporphyrin IX (Fe (III)PPIX) [4]. The connections between propionate group of porphyrin and Fe (III) center from another group and hydrogen bonds dimer structures are generated, the process known as “Heme polymerization”. In this process, the parasite uses the proper enzymatic method as the result of which soluble heme sheets are converted to insoluble structures called hemozoine. The hemozoine (β-hematin) is the critical target for the treatment of malaria in all the genus of malaria parasite [4,5].
Different antimalarial drugs such as chloroquine (CQ), Doxycycline, malaron (atovaquone, proguanil), mefloquine, primaquine, clindamycin, coartem (artemether and lumefantrine), and artesunate are believed to suppress the parasite by inhibiting hematin crystallization [6-8]. According to the latest research, several scaffolds have been evaluated for β-hematin inhibition and antimalarial activities. So far, several synthesized compounds have been tested for the inhibition of β-hematin including atovaquones (floxacrin, the aniline acridin derivative, and acridinon derivatives), hydroxypyridinoes [9,10], Thiazolidine-4-one derivatives ring systems (such as thiazolidin-4-one, 2,3-dihydro-benzo[e] [1,3]-thiazin-4-one and [1,3] thiazinan-4-one) as compared with CQ and showed comparable activity. These structures have been potent, and some cases had better IC50 than CQ [11] as shown in Table 2. Quinolones such as CQ can have interactions with porphyrin rings of heme sheets based on electrostatic interaction π-π stacking [12,13]. Drug residence in Plasmodium falciparum has inspired the discovery of antimalarial medicines with different mechanisms [14]. 3-hydroxypyridin-4-ones were reported as new antimalarial drugs recently [15]. Derivatives of these compounds were evaluated based on β-hematin inhibition and different results were obtained after the substitution of groups on aromatic rings [16]. It has been proven that antiplasmodial activity depends on β-hematin inhibition.
Iron chelating compounds such as desferroxamine (DFO) were suggested as hemozoin inhibitor agents, but these drugs possess insufficient absorption and resistance. For this reason, 3-hydroxypyridin-4-ones (HPOs) were designed as iron-chelating agents for the inhibition of malaria parasite growth [17]. HPOs are bidentate ligands to make bonds with iron and porphyrin groups in heme sheet [18]. The first type of these derivatives is deferiprone followed by new HPO derivatives synthesized through the substitution of groups in suitable positions for β-hematin inhibitors [18-20].
The present study aims to pharmacophore analysis, synthesize and screen structures designed based on previous compounds [18,21]; also, some of these derivatives were synthesized based on Schiff’s bases. Schiff’s bases are widely used for organic compounds synthesis, and according to recent studies, the imine group has shown antifungal, antimalarial, and antibacterial features [22-24]. In addition, derivatives of Schiff's bases with aromatic aldehyde or amine groups have proven efficacious in the inhibition of P. falciparum [25]. Based on pharmacophore results as heme binder and computer-aided aspects by qualitative 3D-pharmacophore and at the next step we studied β-hematin inhibition.
Accordingly, the first evidence of the molecular mechanisms of hematin crystallization and antimalarial drug action as crystal growth inhibitors was found. It helped identification and optimization of functional moieties that bind to crystal surface sites, thus providing guidelines for the discovery of novel antimalarial to the increased potency of current drugs.
Material and Methods
All commercially available chemicals were prepared from either Merck or Sigma-Aldrich. Total solvents used without further purification. FT-IR spectra were recorded on Agilent spectrophotometer. 1HNMR and 13CNMR spectra were performed using Bruker 500 MHz spectrometers (Bruker Bioscience, Billerica, MA, USA) and the chemical shifts are reported in ppm related to TMS peak as the internal standard. Mass spectra (MS) data are recorded on an ESI mode Shimadzu. The UV-visible spectra were reported using a CAMAG spectrophotometer. TLC (analytical thin-layer chromatography) was inspected on silica-gel 60 F254 plates. The Sephadex LH20 (Fluka, Germany) was utilized for purifications of compounds. Melting points were obtained on a Büchi Melting Point B-540 apparatus (Büchi Labor technic, Flawil, Switzerland) and uncorrected.
Experimental Procedures
Synthesis 5-(benzyloxy)-2-(hydroxymethyl)-4H-pyran-4-one (2)
To a suspension of kojic acid (2, 10g, 0.06mol) in methanol (100mL), sodium hydroxide solution (10mL) was added (2.79 g, 0.06 mol). Benzyl chloride (8.0mL, 0.06 mol) was poured dropwise to the mixture. The reaction mixture was refluxed for 6 hr. The progress of the reaction was checked by TLC. After completion of the reaction, the solvent evaporated in vacuum to obtain dark brown oil. Substance diluted with 100 mL of water extracted with dichloromethane (3 × 100mL). The organic layer was washed with sodium hydroxide solution 5% (150mL) and dried over anhydrous Mg2SO4. At last, a brown precipitate obtained that was recrystallized from ethyl acetate to obtain 8.6 g product as a needle-like powder with 86% yield and Mp 121-122 ºC (lit: 91%, 120-122 ºC) [26].
Synthesis of 5-(benzyloxy)-2-(hydroxymethyl)-1-methylpyridin-4(1H)-one (3)
To a solution of benzyl kojic acid (2, 10g, 0.043mol) in ethanol (100 mL) was added 40% aqueous solution of methyl amine (1.39g, 0.064 mol) and the mixture was refluxed. Then, the resulted precipitate was separated from the solution by filtration and recrystallized from ethyl acetate to afford the pure product as a white powder (82% yield) and Mp 215-216 ºC (lit: 77%, 218-220 ºC) [26]. 1H-NMR (500 MHz, MeOD, δ ppm: 3.3 (s, CH3OH), 4.9 (H2O)) δ ppm: 3.58 (s, 3H, CH3), 4.36 (s, 2H, CH2), 5.01 (s, 2H, CH2), 6.22 (s, 1H, H-C6 in pyridinone ring), 7.31-7.41 (m, 5H, C6H5), 7.55 (s, 1H, H-C3 in pyridinone ring). MS, m/z: 245 (M+).
Synthesis of 5-(benzyloxy)-1-methyl-4-oxo-1, 4-dihydropyridine-2-carbaldehyde (4)
To a Boiling solution of 1, 4-dioxan (500 mL) and compound 3 (10g, 0.041 mol) was added MnO2 (70g). Then, the mixture was refluxed, and the progress of the reaction was monitored by TLC. After the reaction was completed (20 min) the MnO2 was filtered off and the solvent was evaporated in vacuum to yield a pale brown solid. Finally, the compound was recrystallized from ethyl acetate to give 7.0 g the pure product as a pale-yellow solid with 76% (7.0 g) yield and Mp 172-174 ºC. IR (KBr): 1437 (C=C, aromatic, bend), 1583 (shouldered, C=C aromatic, C=C alkene), 1618 (C=O ketone,str), 1716 (C=O, aldehyde, str), 2893 (C-H, aliphatic, str),3047 (C-H, aromatic, str). 1H-NMR (500 MHz, MeOD, δ ppm: 3.3 (s, CH3OH), 4.9 (H2O)) δ ppm: 3.58 (s, 3H, CH3), 5.01 (s, 2H, CH2), 6.19 (s, 1H, H-C6 in pyridinone ring), 7.31-7.50 (m, 5H, -C6H5), 7.49 (s, 1H, H-C3 in pyridinone ring), 9.68 (s, 1H, -COH). MS, m/z: 243 (M+).
General Procedure for The Synthesis Of 5a-C Compounds
To a solution of compound 4 (0.01 mol) in 30 mL of absolute ethanol, was added aniline (0.01 mol) dropwise and the reaction mixture was refluxed. The final compound was evaporated in vacuum. The solid compound was prepared to the other procedures.
Synthesis of (E, Z)-5-(benzyloxy)-1-methyl-2-((phenylimino) methyl) pyridin-4(1H)-one (5a)
To a solution of 5-(benzyloxy)-1-methyl-4-oxo-1,4-dihydropyridine-2-carbaldehyde (4, 27.8 g, 0.22 mol) in absolute ethanol (30 mL) was added aniline (0.01 mol,1 mL) dropwise. Then, the reaction mixture was refluxed. When the reaction was completed, the solvent was evaporated in vacuum to yield a light brown solid. The resulted compound was recrystallized from ethyl acetate. For further purification, sefadex column chromatography was used (eluent: methanol, Rf = 0.63) to give a yellow pure product with 61% (16.7 g) yield and Mp 180-181 ºC). IR (KBr): 1485 (C=C, aromatic, bend), 1527 (C=C, aromatic, str.), 1571 (C=N, str.), 1631 (C=O, str.), 2968 and 2924 (C-H, aliphatic, str.), 3059 (C-H, aromatic, str.). 1H-NMR (500 MHz, MeOD, δ ppm: 3.3 (s, CH3OH), 4.9 (H2O)) a, b isomers: a/b= 12%/ 88%, δ ppm: 3.75 (s, 3H, N1-CH3 (a)), 4.08 (s, 3H, N1-CH3 (b)), 5.1 (s, 2H, O-CH2 (a)), 5.2 (s, 2H, O-CH2 (b)), 6.73-7.5 (m, 12H, OCH2-Pheny-H (a, b), N-Phenyl-H (a, b), HC3-H (a), HC6- H (b)), 5.67 (s, HC6- H (a)), 7.63 (s, HC3-H (b)), 7.7 (s, H, N=CH (a)), 8.6 (s, H, N=CH, (b)). 13C NMR (500 MHz, MeOD) δ ppm: 40.09, 41.65, 70.92, 82.50, 113.47, 113.62, 118.71, 120.98, 122.44, 126.49, 127.31, 127.63, 127.66, 127.71, 127.81, 127.82, 127.91, 127.95, 127.14, 127.18, 128,19, 128.64, 128.86, 129.03, 129.07, 129.19, 129.20, 129.35, 136.14, 136.34, 142.73, 143.60, 144.64, 145.55, 146.16, 147.76, 148.74, 150.23, 151.88, 152.67. MS, m/z: 318 (M+).
Synthesis of 5-(benzyloxy)-1-methyl-2-((p-tolylimino) methyl) pyridin-4(1H)-one (5b)
An identical reaction procedure as like that of 5a was used but in this case methyl aniline (0.01mol, 1.07g) was used in place of aniline. The resulting compound was purified by sefadex column chromatography (eluent: methanol, Rf : 0.55) to give the pure product with yellow color with 62% (16.7 g) yield and Mp: 151- 152 ̊C. IR(KBr): 1485 (C=C, aromatic, bend), 1527 (C=C, aromatic, str.), 1570 (C=N, str.), 1631 (C=O, str.), 2924 and 2968 (C-H, aliphatic, str.) 3059 (C-H, aromatic, str.). 1H-NMR (500 MHz, MeOD, δ ppm: 3.3 (s, CH3OH), 4.9 (H2O)) a, b isomers: a/b= 54%/ 46%, δ ppm: 2.2 (s, 3H, Phenyl-CH3 (a)), 2.35 (s, 3H, Phenyl-CH3 (b)), 3.77 (s, 3H, N1-CH3 (a)), 4.05 (s, 3H, N1-CH3 (b)), 5.08 (s, 2H, O-CH2 (a)), 5.15 (s, 2H, O-CH2 (b)), 6.7-7.47 (m, 12H, OCH2-Pheny-H (a, b), N-Phenyl-H (a, b), HC3-H (a), HC6-H (b)), 5.6 (s, HC6-H (a)), 7.6 (s, HC3-H, (b)), 7.73 (s, H, N=CH (a)), 8.6 (s, H, N=CH, (b)). 13C NMR (500 MHz, MeOD) δ ppm: 19.16, 19.75, 40.10, 41.62, 70.93, 82.86, 95.86, 113.64, 116.07, 121.10, 127.64, 127.67, 127.70, 127.81, 127.82, 127.89, 127.95, 127.96, 128.13, 128.17, 129.00, 129.09, 129.10, 129.17, 129.30, 129.43, 129.48, 129.57, 136.14, 136.33, 137.79, 140.42, 142.20, 142.87, 143.13, 146.00, 147.09, 147.28, 147.46, 147.78, 150.52, 151.11, 151.30. MS, m/z: 332 (M+).
Synthesis of 5-(benzyloxy)-2- ((4-ethylphenyl) imino) methyl)-1-methylpyridin-4(1H)-one(5c)
The procedure was described for synthesis of 5a that except 4-Ethyl aniline (0.01 mol, 1.21 g) was used instead of aniline in the reaction mixture. The resulting compound was purified by sefadex column chromatography (eluent: methanol, Rf : 0.61) to give a yellow pure product with 62% (16.7 g) yield, Mp 158- 159 ̊C. IR (KBr): 1485 (C=C, aromatic, bend), 1527 (C=C, aromatic, str.), 1577 (C=N, str.), 1631 (C=O, str.), 2924 and 2958 (C-H, aliphatic, str.), 3059 (C-H, aromatic, str.).1H-NMR (500 MHz, MeOD, δ ppm: 3.3 (s, CH3OH), 4.9 (H2O)) a, b isomers: a/b= 58%/ 42%, δ ppm: 1.15 (t, J=10 Hz, 3H, Phenyl-CH2- CH3 (a)), 1.25 (t, J=10 Hz, 3H, Phenyl-CH2-CH3 (b)), 2.5 (q, 2H, J=10 Hz, Phenyl-CH2-CH3 (a)), 2.68 (q, J=10 Hz, 2H, Phenyl-CH2-CH3 (b)), 3.75 (s, 3H, N1-CH3 (a)), 4.08 (s, 3H, N1-CH3 (b)), 5.1 (s, 2H, O-CH2 (a)), 5.15 (s, 2H, O-CH2 (b)), 5.6 (s, HC6-H (a)), 6.7-7.5 (m, 12H, OCH2-Phenyl-H (a, b), N-Phenyl-H (a, b), HC3-H (a), HC6-H (b)), 7.6 (s, HC3H (b)), 7.68 (s, H, CH=N (a)), 8.62 (s, H, CH=N (b)). 13C NMR (500 MHz, MeOD) δ ppm: 14.77, 15.18, 27.64, 28.09, 40.12, 41.63, 70.92, 82.82, 93.30, 95.86, 113.58, 113.66, 113.72, 113.92, 115.10, 116.13, 121.19, 127.63, 127.67, 127.68, 127.69, 127.81, 127.89, 127.93, 128.03, 128.113, 128.14, 128.17, 128.43, 129.08, 129.16, 129.20, 134.74, 136.14, 136.34, 136.57, 142.86, 143.34, 144.23, 144.94, 147.27, 147.69, 151.43, 152.87. MS, m/z: 346 (M+).
Synthesis of 5-hydroxy-1-methyl-2-((phenylamino) methyl) pyridin-4(1H)-one (6a)
Compound 5a (0.0012mol, 0.4g) was dissolved in 20 mL ethanol and subjected to hydrogenation for 2 hr in the presence of 5% Pd/C catalyst. After that, the product was filtered, and the solvent was evaporated to give a yellow solid. Recrystallization of resulting compound from ethyl acetate yielded a white powder, (0.34 g, 85% yield) %); Mp: 235-237 ºC. IR(KBr): 1311 (C-N, str), 1450 (C=C, aromatic, bend), 1562 (C=C, aromatic, str), 1604 (C=O, str.), 2924 (C-H, aliphatic, str.), 3321 (N-H, str.), 3394-3600 (O-H, str). 1H-NMR (500 MHz, MeOD, δ ppm: 3.3 (s, CH3OH), 4.9 (H2O)) δ ppm: 3.7 (s, 3H, N1-CH3), 4.2 (s, 2H, CH2-NH), 6.48 (s, 1H, HC6-H), 7.45 (s, 1H, HC3-H), 6.57-7.02 (m, 5H, NH-Phenyl-H). 13C NMR (500 MHz, MeOD) δ ppm: 39.68, 43.8, 103.13, 112,34, 115.48, 117.18, 128.14, 128,78, 131.26, 139.43, 142.03, 147.6, 187,36. MS, m/z: 230 (M+).
Synthesis of 5-hydroxy-1-methyl-2-((p-tolylamino) methyl) pyridin-4(1H)-one (6b)
The procedure was described for synthesis of 6a but in this case 5b (0.0012mol, 0.4g) was used instead of 5a in the reaction mixture. Recrystallization from ethyl acetate gave the desired product as a white powder in 83% yield (0.33 g), Mp 242- 243 ºC. IR (KBr): 1319 (C-N, str), 1462 (C=C, aromatic, bend), 1527 (C=C, aromatic, str), 1635 (C=O, str.),2920(C-H, aliphatic, str.), 3332 (N-H, str.), 3402-3600 (O-H, str). 1H-NMR (500 MHz, MeOD, δ ppm: 3.3 (s, CH3OH), 4.9 (H2O)) δ ppm: 2.19 (s, 3H, Phenyl-CH3), 3.8 (s, 3H, N1-CH3), 4.3 (s, 2H, CH2-NH), 6.5 (s, HC6-H), 6.55 (d, J=5 Hz, 2H, HC6'-H, HC2'-H), 6.92 (d, J=10 Hz, HC3'-H, HC5'-H), 7.5 (s, HC3-H). 13C NMR (500 MHz, MeOD) δ ppm: 19.09, 39.70, 44.16, 95.85, 112.55, 112.82, 112.95, 126.24, 126.34, 129.19, 145.29, 146.84, 147.54, 171.03. MS, m/z: 244 (M+).
Synthesis of 2- ((4-ethylphenyl) amino) methyl)-5-hydroxy-1-methylpyridin-4(1H)-one (6c)
The procedure was described for synthesis of 6a as the same for synthesis of 6c. 5c (0.0011 mol, 0.4 g) was used in place of 5a in the reaction mixture. Recrystallization from ethyl acetate gave the desired product as a white powder in 80% yield (0.32 g), Mp decompose at 200 ºC. IR(KBr): 1319 (C-N, str), 1462 (C=C, aromatic, bend), 1523 (C=C, aromatic, str), 1566 (N-H, str.), 1635 (C=O, str.), 2924(C-H, aliphatic, str.), 3302 (N-H, str.), 3417-3600 (O-H, str). 1H-NMR (500 MHz, MeOD, δ ppm: 3.3 (s, CH3OH), 4.9 (H2O)) δ ppm: 1.15 (t, J=10 Hz, 3H, Phenyl-CH2-CH3), 2.5 (q, J=10 Hz, 2H, Phenyl-CH2-CH3), 3.73 (s, 3H, N-CH3), 4.25 (s, CH2-NH), 6.52 (s, HC6-H), 6.6 (d, 2H, HC6'-H, HC2'-H), 6.95 (d, HC3'-H, HC5'-H), 7.38 (s, HC3-H). 13CNMR (500 MHz, MeOD) δ ppm: 22.85, 27.60, 39.56, 44.21, 95.86, 112.59, 115.96, 126.55, 127.28. 128.03, 128.33, 128.83, 133.12, 145.61, 178.98. MS, m/z: 258 (M+).
Biological Assay Method
β -Hematin Assay Method
The biological assay was performed by ITHD (Inhibition test of heme detoxification) method which previously was described by Huy et. al [27] with sight modification [28]. The procedure of this test was as follows: hemin chloride was dissolved in DMSO that diluted to 60 µg/ mL by sodium acetate buffer (1M, pH=4.5), tween 20 was diluted to 0.012 g/ L with distilled water and synthesized compounds were solved by DMSO. Hemin, tween 20 and samples were distributed in each well of a 96-well plate with a ratio of 9:9:2 respectively in triplicate. The final concentration of all the samples in each well was 200 µg/ mL.
In this test, we were prepared a control in triplicate under the same condition in the absence of hemin. This controls allowed being prevented interferer absorption that remains from the matrix sample. In the negative control, we dissolved samples in DMSO without hemin and used chloroquine phosphate as a positive control. The plates were incubated at 60oC for 24h. Additionally, the materials in plates were mixed with micropipette and then adsorption of the solutions was read by ELISA (Epoch, BioTeK, USA) that was performed at 405 nm.
Molecular Docking Studies
All the software and related computational procedures in this research were performed in Marvin Sketch [21], GOLD and Accelry Discovery Studio 4.1 (DS) [29]. The genetic optimization for ligand docking (GOLD) (CHRMm-based forcefield) was carried out to investigate the binding mode of synthesized compounds with heme to the selected active site. GOLD is a CHARMm-based docking tool [30]. Structures of ligands were created via the Chem Draw program and were transformed into DS. The partial charges of compounds were calculated by the Momany–Rone option were typed with CHARMm force field [31]. The energy resulting structures were minimized through Smart Minimizer which performs 1000 steps of steepest descent with a RMS gradient tolerance of three, followed by Conjugate Gradient minimization [32]. X-ray crystallographic structure was downloaded in the PDB entry 5E29 which presented in RCSB protein data bank (http://www.pdb.org) [33]. Then protein preparation and ligand minimization were performed by Discovery Studio. Before docking procedure, Heme sheet removed from hemoglobin then put in a new page and a sphere binding site approximately 8.5 A˚ radiuses were defined around it to confirm atoms of the ligand and the atoms within the sheet. Other parameters were set with the help of gold protocol settings. Also, 10 conformations were considered for each ligand then the best-docked conformation based on gold score fitness (negative value interaction energy plus ligand strain) is applied to rank the poses of every input ligand [30].
Pharmacophore Model
A pharmacophore is an ensemble of essential molecular features that is necessary for biological activity [34,35]. The ‘scaffold hopping’ concept initiated from computational chemistry. It mentions to the search for compounds that have similar activity but contain different core structures. In addition, activity, other molecular properties might be measured. The goal of scaffold hopping is the identification of structurally diverse compounds that are similar in activity and chemical space. Common features based qualitative hypothesis (Hip Hop) is one of hypothesis generation methods that have been successfully used in drug discovery research [36]. It generates and ranks the hypotheses that correlate best three-dimensional arrangements of chemical features with the biological activity variations in the bioactive compounds. In the next step, the most probable features for the acridinone derivatives were selected with considering the chemical.
Nature of the compounds and common feature pharmacophore protocol that was generated using only most active compounds and was predicted to qualitative model. Pharmacophore model was then generated by using ‘common feature pharmacophore generation’s protocol” and the top 10 scoring hypotheses were exported. All the features in the developed pharmacophore models are equal in weight and have the same contribution to predicting the activity of the molecule [37].
ADMET and Properties Predictions
ADMET prediction module of the Discovery Studio (4.1 Accelry clients) were used for evaluating the pharmacokinetics profiles of the new synthesized ligands. Molecular descriptors were used for calculating the drug pharmacokinetics and toxicity for ADMET prediction. The prediction results and plot are described in the results and discussion.
Results and Discussion
Inhibition of the formation β-hematin is an important target for the treatment of malaria disease. Previous studies have shown hydroxypyridinones and Schiff's bases with the aromatic structure are known to iron chelator and antimicrobial thus these compounds may utilize to limit parasite proliferation [17,23]. As mentioned, the compounds with aromatic structures can be influence duo to non-covalent interaction with porphyrin rings of heme for inhibiting of β-hematin formation according to molecular docking results.
Chemistry
The 2-aniline hydroxypyrydinone derivatives with benzyl and hydroxyl group in position 5 were prepared as target compounds. As shown in Scheme 1, the aldehyde compound 4 obtained from a three-step reaction. At first, the hydroxyl group of kojic acid (at position 5) was protected by benzyl group. In the second step, pyridinon ring was obtained via reaction of benzylated kojic acid with methylamine.
The Michael reaction is the initial reaction for conversion pyranone ring to pyridinone by ring-opening and ring closure. In this reaction, β-carbon has electron deficiency because of Mesomerisation of α, β-unsaturated carbonyl compound therefore susceptible to nucleophilic attack. When the primary amine attacks at both α, β-unsaturated functions as a nucleophile leads to the conversion of the pyranone ring to pyridinone with the loss of a H2O molecule. Protection of the hydroxyl group in position 5 has critical role in this reaction. It is believed that the unprotected hydroxyl group undergoes a Michael reaction with intermediates formed during the amination step. Further condensation products were caused in more consumption of kojic acid and therefore lead to low yield. Oxidation of hydroxyl group at 2 positions of benzylated hydroxyoyridinone ring by activation with Manganese dioxide (MnO2) in 1,4-dioxan gave the corresponding aldehyde [4]
(Scheme 1).
The prepared aldehydes were used as intermediate for the preparation of some imine derivatives of hydroxypyridinone (Schiff base). Schiff bases are general class of compounds that known as imines or azomethines. The general schiff base structure is RN=CR'R'' that R' and R'' can be alkyl, aryl, heteroaryl, or cycloalkyl [38]. These structures formed as condensation products from primary amines (aromatic or aliphatic) and aldehyde or ketone group according to last studies. In the condensation procedure, aldehyde group is reacted faster than ketone group as outlined in scheme 2 [39]. Primary amines are used as the nucleophile group that attack to the carbonyl group in aldehyde. It was found that the appropriate solvent and catalytic condition for this reaction are ethanol and acetic acid, respectively. Previous studies have shown that the synthesized compounds of schiff bases usually have achieved high yields.
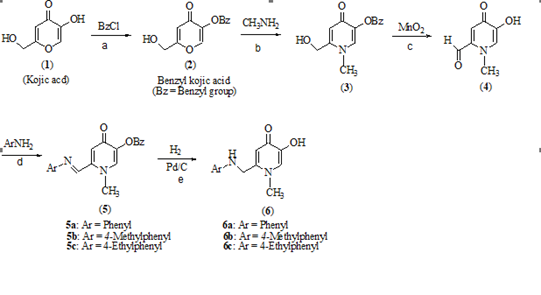
Scheme 1: Synthesis of 1-substituted-3-hydroxypyridin-4-one’s derivatives, reagent, and conditions: a) reflux in methanol; b) reflux in ethanol and water; c) reflux in 1,4-dioxane; d) reflux in absolute ethanol; e) stirrer in ethanol at room temperature.
The electrophilic carbon and nucleophilic nitrogen properties of imine (-N=C-) form that have provided binding interaction with different electrophiles and nucleophiles. Nitrogen groups were showed Lewis’s base functional so that it can coordinate with metal ions in different structures as donor electron and act as an effective inhibitor so that nitrogen atom in amine structures, was reacted the same method [40]. The presence of an aromatic conjugation system and the schiff bases of aromatic substitution of aldehydes with amines were revealed more stability than aliphatic substitution. Therefore, the aromatic primary amine (aniline derivatives) was used for the synthesis of 5a-5c with schiff bases reaction. These imine compounds have isomer structure containing E and Z isomers. Each of these isomers has been clear in the different percentages. The corresponding peaks of each isomer have been appeared at the 1HNMR and FTIR spectrums and are match with the results reported in the recent studies [41], Finally, debenzylation of the related imine compounds (5a-5c) afford to the desired final hydroxypyridinones (6a-6c). Interestingly, based on spectra characterization, not only the hydrogenation process was used to remove the benzyl group but also the double bond in the imine derivatives (N=C) converted to single bond such as amine compounds (N-C) (Scheme 2).
Molecular Docking Analysis
Molecular Docking Study of Hit Compounds with Best Scores
In this study, gold software was used as an accurate and powerful docking system to molecular docking simulation. Also, the analysis of the desired compounds was performed by Discovery Studio 4.1 and minimized by CHARMm force field. For biological evaluations using docking, numerically arranged G-score values were considered that indicate the interactions between the heme sheet and the desired compounds.
In the early stages of docking, the greatest focus was on the specific connections of the synthesized compounds, and heme sheet based on topographic features or energy values, and all the docking runs for each molecule were evaluated and analyzed to increase power and accurately to identify best interactions of synthesized compounds. Although the presence of heme-ligand complex crystallography is a challenge, finding a reasonable and acceptable mechanism for inhibition of β-hematin by finding the interactions between the heme sheet and the desired ligands is essential; therefore, attempts have been made to remove the heme sheet from the hemoglobin crystallography. So that, to ensure the docking results using gold software, the docking results of the synthesized ligand complex and heme sheet were compared with both the chloroquine-heme complex, which had the potential to inhibition of β-hematin formation in biological results (Figure 1).
Molecular Docking Study of The Potent Compound Complex with Heme Sheet
Among the synthesized compounds, we introduced 5b as the compound with the highest G-score fitness (Table 1). Validation of its G-score fitness associate with hydrogen-bonding, aromatic, and lipophilic interactions were identified by biological assay and chloroquine interaction. Based on the docking results, 5b was considered as potent compound in β-hematin inhibition due to strong interactions inside active sites of the heme sheet compared with CQ binding mode in active site as the positive control [12,42].
The present study by gold states that; the benzyl group and aromatic derivatives in positions 5 and 2, justify strong interactions such as π-alkyl, π-π stacking, Fe…N axial interaction, and alkyl interactions for activation between the target ligand (5b) and heme sheet [21] (Figure 1). Consequently, existence of compatibility the non-covalent interactions between this class of synthesized compounds and heme sheet can help design a pharmacophore scaffold in the synthesis of antimalarial compounds [21,42].
In this study, we investigated the role of substitution of aniline derivatives in position 2, and phenyl and hydroxide groups in position 5. The structure activity revealed that the porphyrin ring in the heme sheet has an effective overlap with the combination of 5b that was like the interaction between CQ and the heme sheet as docking has shown, so that their results of biologic could similar (Table 1&3). Surprisingly, despite the replacement of the methyl group with hydrogen on the aniline ring and the multiplication of biological effect, increasing the length of the alkyl chain in terms of carbon number on the ring did not have a positive effect on further inhibition of β-hematin formation. According to CQ structure and Cl group, it can be found that the presence of halogen groups on aromatic structures can inhibit β-hematin. Also, the effectiveness of aromatic groups for antimicrobial activity and impressive efficiency of the benzyl group instead of the hydroxyl group in position 5 in the active site duo to the non-covalent interaction and hydrogen bonds between it and hydrophobic groups were identified. The porphyrin rings in the sheet that close to the pharmacophore results of acridinone derivatives based on the docking and pharmacophore results contributed to the effectiveness of imine structures, the 5b compound, to inhibit β-hematin compared to amino structures. It should be noted that this study has investigated the effectiveness of these compounds to inhibit β-hematin among other ways to treat and control malaria (Table 3).
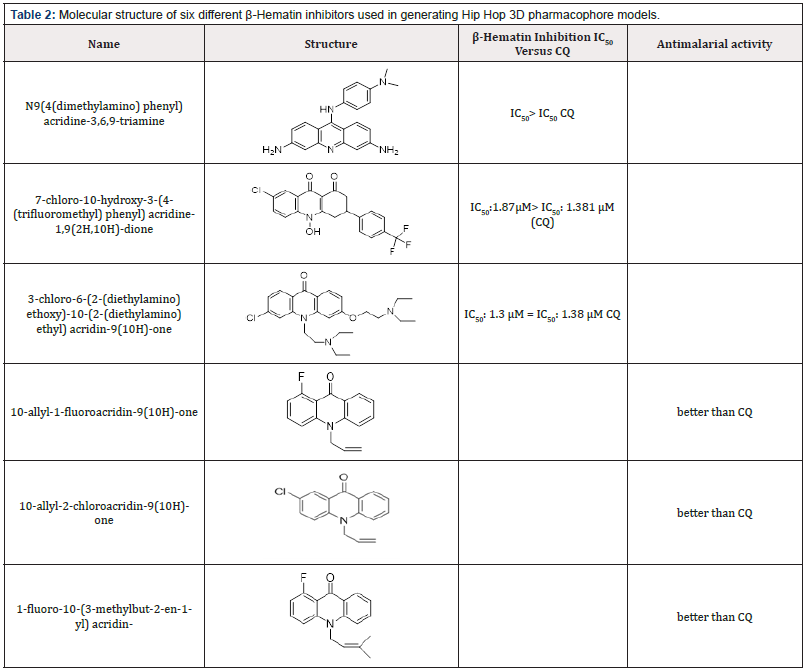
Table 2: Molecular structure of six different β-Hematin inhibitors used in generating Hip Hop 3D pharmacophore models.
3D Pharmacophore Modeling
The pharmacophore analysis for β-Hematin inhibitors in six different structures (Table 2) has shown three essential features, including H bond acceptor and hydrophobic properties with specific radius (Figure 3). The HipHop pharmacophore models were generated by considering the principal value 2 for all ligands and maximum omitted feature was 0. The final HipHop models, according to common 3D pharmacophore features HHA, were ranked. The interaction patterns generated by 3D pharmacophore models were compared to the interaction modes extracted by molecular docking and newly synthesized compounds with the biological assay. In this investigation, it was shown that the contribution of hydrophobic features was determinant of the activity or inactivity of a typical ligand (Table 1&2) (Figure 2&3).
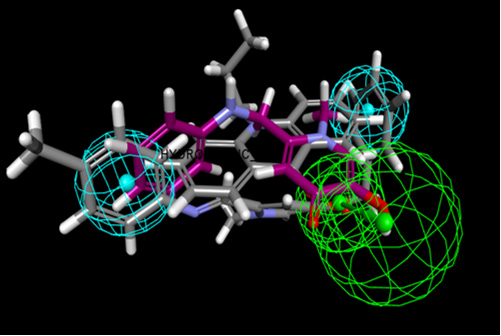
Figure 2: Generation of the best 3D-HipHop pharmacophore model. H-bond acceptor groups (green), hydrophobic groups (blue).
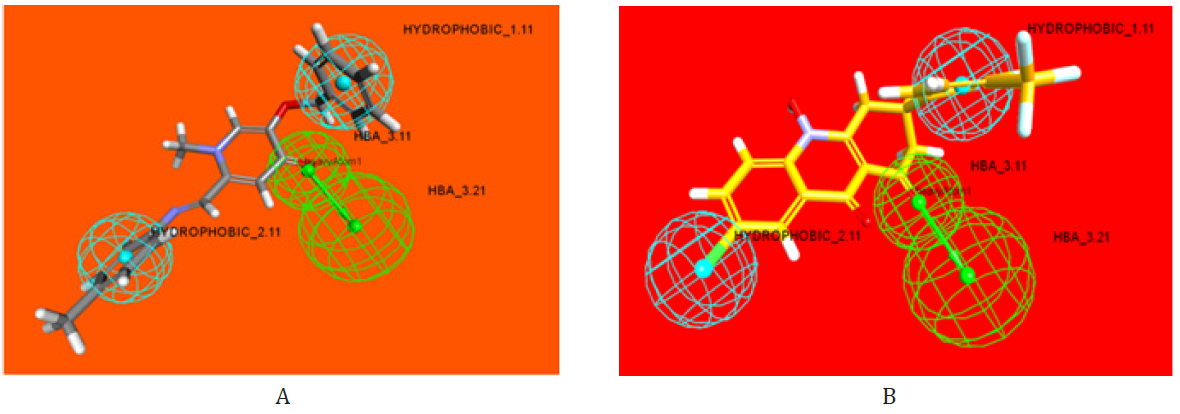
Figure 3: Generation of the best 3D-HipHop pharmacophore model of the most active synthesized ligand pharmacophore
A) The previous active compounds.
B) H-bond acceptor groups (green), hydrophobic groups (blue).
ADMET Evaluation
Most new small molecules candidate fail because of their poor ADMET profiles. There are several in silico methods for predicting the pharmacokinetics profile of a molecules and parameters were evaluated, by consideration Lipinski's "Rule of Five" [43]. All calculated parameters for the novel synthesis molecules were well within the prescribed ranges. The ADME screening results are summarized in Figure 4. The blood-brain barrier penetration (BBB) and 2D PSA are inside the 95% and 99% confidence ellipses. The screening results of ADME model showed that all synthesized compounds possess a 99% confidence level for human intestinal absorption and blood-brain barrier (BBB). The AlogP was associated with absorption or penetration; therefore, the value should be less than 5. The PSA is another item property correlated to drug bioavailability that should be smaller than 140 [44] (Figure 4).
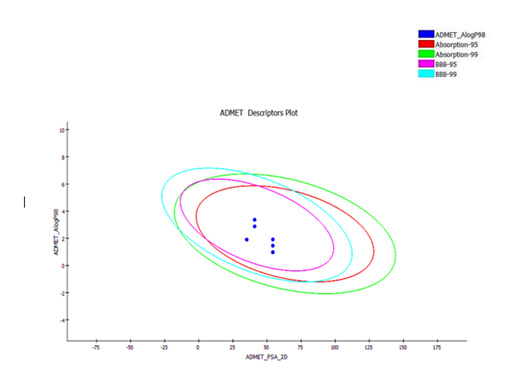
Figure 4: ADMET properties predicted for synthesized compounds; that located inside the innermost oval and had the best results.
Structure Activity Relationship
This study has shown the effect of substitution of aniline derivatives in position 2, phenyl and hydroxide groups in position 5. Structure activity relationship revealed the porphyrin ring in the heme sheet have an effective overlap with the combination of 5b that was similar to the interaction between CQ and heme sheet as docking has shown, so that their results of biologic could similar (Table 1). These results have shown despite the replacement of the methyl group with hydrogen on the aniline ring and the multiplication of biological effect, increasing the length of the alkyl chain in terms of carbon number on the ring, contrary to expectations, did not have a positive effect on further inhibition of β-hematin formation. According to CQ structure and Cl group can be found that the presence of halogen groups on aromatic structures can be effective in inhibiting β-hematin using this biological technique. Also, the effectiveness of aromatic groups for antimicrobial activity and impressive efficiency of the benzyl group instead of the hydroxyl group in position 5 in the active site due to non-covalent interaction and hydrogen bonds between it and hydrophobic group was recognized. The iron chelating structure such as 6a-c without benzyl group was decreased inhibition activity because of geometry of ligands is important to best overlapping of heme sheet. The best activity for iron chelating agent was identified to scavenge free heme in malaria disease [15,17].
The porphyrin rings in the sheet that close to the pharmacophore results of acridinone derivatives as antimalaria compounds that have reported higher activity than CQ based on the docking and pharmacophore results caused the effectiveness of imine structures 5b compound to inhibit β-hematin compared to amino structures. The previous effective structures such as acridinone derivatives were used to compare and validate theoretical results and new results of interaction mode, pharmacophoric features of new scaffold that was presented in this investigation. Repurposing and previous pharmacophore evaluation approaches were considered to find new potential leads against new pandemic treatment and several resistance diseases such as cancer [44-46]. It should be noted that studies have shown different ways to treat malaria, and these compounds were evaluated only to inhibit β-hematin in this study and may be used in other ways for control and treatment diseases.
Conclusion
In this study, the pharmacophore evaluation of new scaffolds and synthesis based on pharmacophore modeling such as hydroxypyridinone derivatives provide an opportunity to predict new effective scaffolds. we investigated the effect of hydroxypyridinones with aromatic derivatives on the inhibition of β-hematine. The mechanism in the novel compound 5b was similar to that of the CQ. Also, the synthesized compounds were evaluated in vitro based on ITHD method. These compounds, based on hydroxypyridinone, have low toxic and acceptable pharmacokinetic ranges. The obtained results showed that these structures can mediate overlap with the heme sheet and potentiate to inhibit β-hematine. Other imine derivatives with aromatic structures may result in better antimalarial effects through the inhibition of β-hematine. Future studies are recommended to design novel structures with antimalarial activities and increase the potency of compounds with aromatic substitution and hydrogen bond acceptor substitution with higher radius based on HBA pharmacophoric features. This method and results can be useful in the discovery of novel compound to treat resistance disease such as malaria.
Acknowledgments
We would like to show our gratitude to the research council of medicinal chemistry department school of pharmacy and pharmaceutical sciences of Isfahan University, we would appreciate traditional medicine and materia medica research centre for their assistance during this research. Grant number: 397309.
Author Contribution
In this article, Dr Fayyazi was contributed to conception, writing, revised and molecular modeling, Mrs. Allahi was contributed as the first authors equally and synthesis, Dr Esmaeili was contributed to biological assay, Prof. Saghaei was contributed to conception, revised and Funding and Dr Rostami was contributed to advise in some procedures.
References
- Penna Coutinho J, Cortopassi WA, Oliveira AA, França TCC, Krettli AU, et al. (2011) Antimalarial activity of potential inhibitors of Plasmodium falciparum lactate dehydrogenase enzyme selected by docking studies. PloS one 6(7): e21237.
- Snow RW, Guerra CA, Noor AM, Myint HY, Hay SI, et al. (2005) The global distribution of clinical episodes of Plasmodium falciparum malaria. Nature 434(7030): 214.
- B Ghasemi J, Shiri F, Pirhadi S, Heidari Z (2015) Discovery of new potential antimalarial compounds using virtual screening of ZINC database. Combinatorial chemistry & high throughput screening 18(2): 227-234.
- Combrinck JM, Joanne E, Hearne GR, Marques HM, Ntenteni S, et al. (2002) Fate of haem iron in the malaria parasite Plasmodium falciparum. Biochemical journal 365(2): 343-347.
- Pagola S, Stephens PW, Bohle DS, Kosar AD, Madsen SK (2000) The structure of malaria pigment β-haematin. Nature 404(6775): 307-310.
- Organization WH (2015) Guidelines for the treatment of malaria: World Health Organization.
- Assessment W (2003) Monitoring of antimalarial drug efficacy for the treatment of uncomplicated falciparum malaria (WHO/HTM/RBM/2003.50). Geneva: World Health Organization.
- Fitzroy S-M, Gildenhuys J, Olivier T, Tshililo NO, Kuter D, et al. (2017) The effects of quinoline and non-quinoline inhibitors on the kinetics of lipid-mediated β-hematin crystallization. Langmuir 33(30): 7529-7537.
- Valdés AFC (2011) Acridine and acridinones: old and new structures with antimalarial activity. The open medicinal chemistry journal 5:11-20.
- Fernández Calienes A, Pellón R, Docampo M, Fascio M, D’Accorso N, et al. (2011) Antimalarial activity of new acridinone derivatives. Biomedicine & Pharmacotherapy 65(3): 210-214.
- Solomon VR, Haq W, Srivastava K, Puri SK, Katti S, et al. (2007) Synthesis and antimalarial activity of side chain modified 4-aminoquinoline derivatives. Journal of medicinal chemistry 50(2): 394-398.
- Egan TJ (2006) Interactions of quinoline antimalarials with hematin in solution. Journal of inorganic biochemistry 100(5-6): 916-926.
- Cohen SN, Phifer KO, Yielding KL (1964) Complex formation between chloroquine and ferrihaemic acid in vitro and its effect on the antimalarial action of chloroquine. Nature 202(4934): 805-806.
- Ismail FM, Drew MG, Navaratnam S, Bisby RH (2009) A pulse radiolysis study of free radicals formed by one-electron oxidation of the antimalarial drug pyronaridine. Research on chemical intermediates 35(4): 363-377.
- Dehkordi LS, Liu ZD, Hider RC (2008) Basic 3-hydroxypyridin-4-ones: potential antimalarial agents. European journal of medicinal chemistry 43(5): 1035-1047.
- Saghaie L, Sakhi H, Sabzyan H, Shahlaei M, Shamshirian D, et al. (2013) Stepwise MLR and PCR QSAR study of the pharmaceutical activities of antimalarial 3-hydroxypyridinone agents using B3LYP/6-311++ G** Medicinal Chemistry Research 22(4): 1679-1688.
- Andayi WA, Egan TJ, Gut J, Rosenthal PJ, Chibale K, et al. (2013) Synthesis, antiplasmodial activity, and β-hematin inhibition of hydroxypyridone-chloroquine hybrids. ACS medicinal chemistry letters 4(7): 642-646.
- Hershko C, Theanacho E, Spira D, Peter H, Dobbin P, et al. (1991) The effect of N-alkyl modification on the antimalarial activity of 3-hydroxypyridin-4-one oral iron chelators. Blood 77(3): 637-643.
- Toso L, Crisponi G, Nurchi VM, Crespo Alonso M, Lachowicz JI, et al. (2013) A family of hydroxypyrone ligands designed and synthesized as iron chelators. Journal of inorganic biochemistry 127: 220-231.
- Toso L, Crisponi G, Nurchi VM, Crespo-Alonso M, Lachowicz JI, et al. (2014) Searching for new aluminium chelating agents: a family of hydroxypyrone ligands. Journal of inorganic biochemistry 130: 112-121.
- Fayyazi N, Esmaeili S, Taheri S, Ribeiro FF, Scotti MT, et al. (2019) Pharmacophore Modeling, Synthesis, scaffold hopping and biological β-hematin inhibition interaction studies for anti-malaria compounds. Current topics in medicinal chemistry.
- Dhar DN Taploo C (1982) Schiff-bases and their applications. Journal of scientific & industrial research 41(8): 501-506.
- Da Silva CM, da Silva DL, Modolo LV, Alves RB, de Resende MA, et al. (2011) Schiff bases: A short review of their antimicrobial activities. Journal of advanced research 2(1): 1-8.
- Ali MA, Haroon CM, Nazimuddin M, Majumder SM-u-H, Tarafder MT, et al. (1992) Synthesis, characterization, and biological activities of some new nickel (II), copper (II), zinc (II) and cadmium (II) complexes of quadridentate SNNS ligands. Transition metal chemistry 17(2): 133-136.
- Krungkrai SR, Krungkrai J (2011) Malaria parasite carbonic anhydrase: inhibition of aromatic/heterocyclic sulfonamides and its therapeutic potential. Asian pacific journal of tropical biomedicine 1(3): 233-242.
- Storr T, Mitchell D, Buglyó P, Thompson KH, Yuen VG, et al. (2003) Vanadyl- Thiazolidinedione combination agents for diabetes therapy. Bioconjugate chemistry 14(1): 212-221.
- Huy NT, Uyen DT, Maeda A, Oida T, Harada S, et al. (2007) Simple colorimetric inhibition assay of heme crystallization for high-throughput screening of antimalarial compounds. Antimicrobial agents and chemotherapy 51(1): 350-353.
- Mosaddegh M, Irani M, Esmaeili S (2018) Inhibition test of heme detoxification (ITHD) as an approach for detecting antimalarial agents in medicinal plants. Research journal of pharmacognosy (RJP) 5(1): 5-11.
- O'Boyle NM, Banck M, James CA, Morley C, Vandermeersch T, et al. (2011) Open Babel: An open chemical toolbox. Journal of cheminformatics 3(1): 33.
- Wu G, Robertson DH, Brooks III CL, Vieth M (2003) Detailed analysis of grid‐based molecular docking: A case study of CDOCKER-A CHARMm‐based MD docking algorithm. Journal of computational chemistry 24(13): 1549-1562.
- Momany FA, Rone R (1992) Validation of the general-purpose QUANTA®2/CHARMm® force field. Journal of computational chemistry 13(7): 888-900.
- Dotolo S, Facchiano A (2017) Pharmacophore modeling, virtual computational screening, and biological evaluation studies. PeerJ preprints 2167-9843.
- Shukla A, Sharma P, Prakash O, Singh M, Kalani K, et al. (2014) QSAR and docking studies on capsazepine derivatives for immunomodulatory and anti-inflammatory activity. PloS one 9(7): e100797.
- Han SY, Choi JW, Yang J, Chae CH, Lee J, et al. (2012) Design and synthesis of 3-(4, 5, 6, 7-tetrahydro-3H-imidazo [4, 5-c] pyridin-2-yl)-1H-quinolin-2-ones as VEGFR-2 kinase inhibitors. Bioorganic & medicinal chemistry letters 22(8): 2837-2842.
- Wang S, Sun H, Liu H, Li D, Li Y, et al. (2016) ADMET evaluation in drug discovery. 16. Predicting hERG blockers by combining multiple pharmacophores and machine learning approaches. Molecular pharmaceutics 13(8): 2855-2866.
- Patil S, Tyagi A, Jose J, Menon KN, Mohan CG, et al. (2016) Integration of common feature pharmacophore modeling and in vitro study to identify potent AChE inhibitors. Medicinal chemistry research 25(12): 2965-2975.
- L'abbate FP, Müller R, Openshaw R, Combrinck JM, de Villiers KA, et al. (2018) Hemozoin inhibiting 2-phenylbenzimidazoles active against malaria parasites. European journal of medicinal chemistry 159: 243-254.
- Dutta B, Bag P, Adhikary B, Flörke U, Nag K, et al. (2004) Efficient proton-templated synthesis of 18-to 38-membered tetraimino (amino) diphenol macrocyclic ligands: structural features and spectroscopic properties. The Journal of organic chemistry 69(16): 5419-5427.
- RJ F, Fessenden JS (1998) Organic chemistry 6th ed california: Brooks/Cole publishing inc pacific grove: Organic chemistry 134-141.
- Hameed A, al-Rashida M, Uroos M, Abid Ali S, Khan KM, et al. (2017) Schiff bases in medicinal chemistry: a patent review (2010-2015). Expert opinion on therapeutic patents 27(1): 63-79.
- Acharya BN, Kaushik MP (2007) Pharmacophore-based predictive model generation for potent antimalarials targeting haem detoxification pathway. Medicinal chemistry research 16(5): 213-229.
- Hameed A, Masood S, Hameed A, Ahmed E, Sharif A, et al. (2019) Anti-malarial, cytotoxicity and molecular docking studies of quinolinyl chalcones as potential anti-malarial agent. Journal of computer-aided molecular design 33(7): 677-688.
- R Li, X Su, Z Chen, W Huang, Y Wang, et al. (2015) Structure-based virtual screening and ADME/T-based profiling for low molecular weight chemical starting points as p21- activated kinase 4 inhibitors, RSC Advances 5: 23202-23209.
- Fayyazi N, Fassihi A, Esmaeili s, Taheri S (2020) Molecular dynamics simulation and 3D-pharmacophore analysis of new quinoline-based analogues with dual potential against EGFR and VEGFR-2. Journal of biological macromolecules 142: 94-113.
- M Saeed, A Saeed, MJ Alam, M Alreshidi (2021) Receptor-Based Pharmacophore Modeling in the Search for Natural Products for COVID-19 Mpro. Molecules 26(6): 1549.
- Fayyazi N, Mostashari-Rad T, Ghasemi JB, Mirabzadeh Ardakani M (2021) Molecular dynamics simulation, 3D-pharmacophore and scaffold hopping analysis in the design of multi-target drugs to inhibit potential targets of COVID-19, Journal of Biomolecular Structure and Dynamics.