Research Article
Creative Commons, CC-BY
Understanding The Role of Mitochondria in The Processes of Differentiation of Mesenchymal Stem/ Progenitor Cells and Male Germ Cells in Case of Male Infertility
*Corresponding author:Iskra Sainova, Department of Pathology and Anthropology with Museum, Institute of Experimental Morphology, Bulgaria.
Received: September 30, 2022; Published: October 18, 2022
DOI: 10.34297/AJBSR.2022.17.002337
Abstract
Differentiation of derived from in vitro-incubated adult human mesenchymal stem cells (MSCs), isolated from bone marrow, cartilage and adipose tissue, respectively, by application of appropriate incubation conditions, to osteoclasts, was investigated and proved. The current results show the importance of the changes in the mitochondrial dynamics about the differentiation of male germ cells (spermatogonia) to mature and functional activity gametes (spermatozoa), as well as of the MSCs in direction osteoclasts and thus, of the receptor activator of tartrate-resistant acid phosphatase (TRAP). In this way, the current results were in agreement with the literature data and confirmed the key role of these organelles in the differentiation direction of stem/progenitor cells. Furthermore, a possibility for the development of new mitochondria-based strategies for the needs of regenerative medicine and assisted reproduction could be proposed.
Keywords:Mesenchymal Stem Cells (MSCs); Cellular Differentiation; Mitochondrial Dynamics; Osteoclasts; Germ Cells; Spermatogenesis
Introduction
Mitochondrial activity, biology and function are major factors that provide normal cellular homeostasis [1,2]. Contemporary technologies allow a deeper observation of the biological characteristics of mitochondria, induced interest in their “behavior” both in vitro and in vivo, as well as determination of mitochondrial genes (e. g., mtDNA), proteins, morphology, and mechanisms that regulate and control the shape, size, number, and distribution of mitochondria (mitochondrial dynamics) [3,4]. They are very plastic cellular organelles that could change their size/shape very quickly (in а matter of seconds) due to their flexible and dynamic inner mitochondrial membrane (IMM). Mitochondria can change their morphology and location in the cell by fusing into larger units or fragmenting (fission), depending on the functional state of the cell type [5]. In rapidly dividing cells, mitochondria are diffused into small spherical fragments in the cytosol (a fragmented form of mitochondria), while in non-dividing cells they are highly interconnected, forming a network (reticular form).
The mitochondrial network is formed by the fusion of individual mitochondria, and the balance between fusion and fission determines the general morphology of mitochondria (plasticity and structure of IMM), which could change dramatically in response to the cellular cycle, intracellular energy levels and cellular stress [6]. Mitochondrial functions are related mainly to the oxidative metabolism of the cells and tissues (including oxidative phosphorylation - OXPHOS and free radicals generation), cellular energy production, as well as processes of cellular proliferation, differentiation, apoptosis, etc. The role of the mitochondria, particularly of their dynamics, has been proposed as essential about the successful differentiation of mesenchymal stem cells (MSCs) [7,8]. MSCs are multipotent stromal cellular progenitors, possessing ability to differentiate into varies directions, including to osteoblasts, osteoclasts, neurons, chondrocytes, myocytes, adipocytes, etc., in dependence of the respective incubation conditions [9].
In a state of maturity, these cellular types show varying degrees of regeneration. The activity level of the mitochondria has been determined as comparatively low in MSCs but increasing on the influence of increased mtDNA copy number, protein levels of respiratory enzymes, oxygen consumption rate, mRNA amounts of mitochondrial biogenesis-associated genes and intracellular ATP content during the differentiation process [10]. In this relation, the role of the mitochondria dynamics has also been proved as underlining the malignant transformation of the stem cells or its escape, respectively [11,12]. However, little is known about the role of the mitochondrial network in osteoclasts derivation. The influence of extracellular matrix (ECM) components as microtubuleassoctiated proteins (MAPs), matrix metalloproteinases (MMPs), mitogen-activated protein-kinases (MAPKs), Nuclear Factor kappaB (NF-κB), as well as nuclear factor-induced kinases (NIKs) and IκB kinases (IKKs) has been proved [13]. Pivotal role of p38α MAPK in the mechanisms, which coordinate osteoclastogenesis and osteoblastogenesis has been established. The influence of NF- κB on the mitochondrial function from both inside and outside the mitochondria, in particular its role in the control of mitochondrial dynamics (fusion and fission) has been proved [14]. As a primary osteoclast differentiation factor, which promotes this process mainly through gene expression control by activating its receptor, has been determined the receptor activator of NF-κB ligand (RANKL) [15]. When RANKL is unable to increase the mtDNA amount, as well as OXPHOS protein expression, lower oxygen consumption rates in mutant osteoclast precursors with lacking NIKs have been assessed, which has confirmed the importance of these organelles about the osteoclast differentiation.
Immunofluorescence confocal microscopy assay has proved co-localization of tartrate-resistant acid phosphatase (TRAP) with RANKL receptor – RANK [16-18]. As another essential factor about cellular osteoclast differentiation has been determined the monocyte/macrophage colony stimulating factor (M-CSF), by binding to its receptor c-Fms, activating the proliferation and survival of osteoclast precursors by distinct signaling pathways. On the other hand, defects in the mitochondrial dynamics, as well as in the balance between fusion and fission processes, have been associated with many diseases and disorders [19]. Morphological studies of the germ cells of patients with various diseases of the reproductive system show a wide range of disorders in these cells, leading to a severe reduction of their fertilizing capacity [20-22]. Investigations of the mitochondria of germ cells in the testes showed that they have different biodynamics and bioenergetic parameters compared to mitochondria in the somatic cells [3,23,24], but have some similarities with stem cells. Mammalian spermatogenesis is a multistage, comprehensive, and prolonged process of differentiation of the germ stem cellular progenitors (spermatogonia) [25] in highly specialized male gametes (spermatozoa) for a determined period (approximately 74 days in humans).
This complex process requires several developmentally regulated metabolic transitions, which are associated with mitochondrial biodynamics, remodeling and plasticity [26,27]. In germ cells during spermatogenesis, specific structural changes in mitochondria occur, which determine three different mitochondrial configurations - orthodox, сondensed and intermediate type. The inter-conversion between the two main morphological configurations (orthodox and сondensed) could be achieved by transformation of the IMM (e. g., cristae formation), which is followed by fusion and/or fission, together with dilatation or condensing of the mitochondrial matrix [28-30]. The orthodox mitochondrial configuration in spermatogonia and spermatocytes in prophase stages (preleptotene, leptotene) is connected predominantly to glycolysis as the main source of ATP [31]. Intermediate and condensed mitochondria in the male germ cells (meiotic spermatocytes and post-meiotic spermatids) during spermatogenesis are preliminary involved in the production of ATP by the OXPHOS mechanism. Enhanced OXPHOS leads also to increased number and size (hypertrophy) of the organelles [32].
The biological characteristics of the mitochondria change dramatically in the process of germ cell differentiation and can serve as biomarkers during the process of mammalian spermatogenesis. In this relation, the main goal of the current study was directed to a better understanding of the mitochondrial biology and dynamics during the differentiation of MSCs to osteoclasts, in particular on the influence of RANKL, as well as to clarify the role of these organelles in the development of male germ cells during spermatogenesis in case of male infertility.
Materials and Methods
Normal MSCs were isolated from human bone marrow, from cartilage and from adipose tissue, and the cell viability was determined by application of eosin dye exclusion test [33]: % cell viability = (total number of viable cells per 1 ml cell suspension/ total number of cells per 1 ml cell suspension) x 100. All cells were seeded at a concentration 3 x 104 cells/cm2. The isolated bone marrow material was subjected to Ficoll-Hypaque density gradient separation. The cartilage tissue was digested by treatment with 0.5% Pronase (Sigma) at 370C for 1 hour, subsequently - with 0.2% Collagenase (Sigma) for 45 minutes, in Dulbecco’s Modified Eagle’s Medium (DMEM) (Sigma) without serum. The adipose tissue was digested by treatment with 0.2% Collagenase (Sigma), in basic Minimum Essential Medium, alpha modification (α-MEM) without serum, at 370C for 45 minutes. After centrifugation, the cellular pellets were resuspended, and the derived stem/progenitor cells were seeded at a density 2x103 - 4x103 cells/ml in basic α-MEM (Sigma), supplemented with FBS (Sigma). In all cases, the MSCs characteristics of the so isolated progenitors are proved previously by flow-cytometry assay: negative on markers CD31, CD34 and CD45, but positive on markers CD90, CD105 and CD106, in opposite of the bone marrow hematopoietic stem cells (HSCs) [34].
For differentiation of the MSCs, isolated from the three sources described, in osteoclasts, method, suggested by Susa et al. (2004), was used [35]. Briefly, after 24-48 hours incubation in basic α-MEM, to separate cellular sub-populations were added 25 ng/ml M-CSF and 50 ng/ml RANKL. The appearance of osteoclasts was observed around the 7-th-10-th day after seeding, by double staining with Hematoxilin and TRAP technique, taking in consideration the proved role of the enzyme TRAP as a marker about osteoclast differentiation because of its established co-localization with the RANKL receptor [16-18]. TRAP activity has been expressed as the hydrolysis of p-nitrophenyl phosphate (pNPP) [36]. In general, after washing the suspension of the derived from the three sources MSCs with PBS (Sigma), they were pre-incubated in the presence of pNPP in its role of substrate of the enzyme TRAP. For confirmation of the observed changes, in vitro-model was also used.
For this goal, mouse embryonic fibroblasts from 3T3 cell line from experimental mice Balb/c were pre-cultivated in cultural fluid from previously incubated in it mouse malignant myeloma cells, after which they were freezed in the presence of cryo-protector Dimethylsulfoxide (DMSO), thawed and reincubated. All cells were cultivated at 370C in thermostat with 5% CO2 and 95% air humidity and observed by inverted light microscope (Leica), supplied with megapixel CCD-camera. For the aim of the present study, fixed microscopy slides about transmission electron microscopy (TEM) were prepared. TEM (“Opton” EM 109 microscope) was used in the study to assess ultrastructural changes in germ cells in a case of male infertility with oligozoospermia and asthenozoospermia. The research was carried out according to the WHO criteria (2010). It is necessary to specify that the case under consideration has a proven diagnosis of male infertility with impaired spermatogenesis and oligozoospermia/asthenozoospermia based on a clinical picture - medical examination/anamnesis, histological studies, semen analysis, and other methods that are not the subject of the present study and are not included therein.
Result
Multi-nuclear TRAP-positive osteoclasts were derived (Figure 1a-1c) from MSCs of the three sources. On the electron micrograph from the used experimental in vitro-model is shown a phase of the fusion between two myeloid-like cells, derived from mouse embryonic 3T3 fibroblasts, after their pre-incubation in cultural fluid from mouse malignant myeloma cells incubation, followed by freesing in the presence of DMSO and re-incubated (Figure 1d). In this separate phase of the fusion process during the osteoclastogenesis could be observed many membrane formations, containing vesicles with different sizes and shapes, as well as parts of the endoplasmatic reticulum (ER) and mitochondria with different types of configurations.
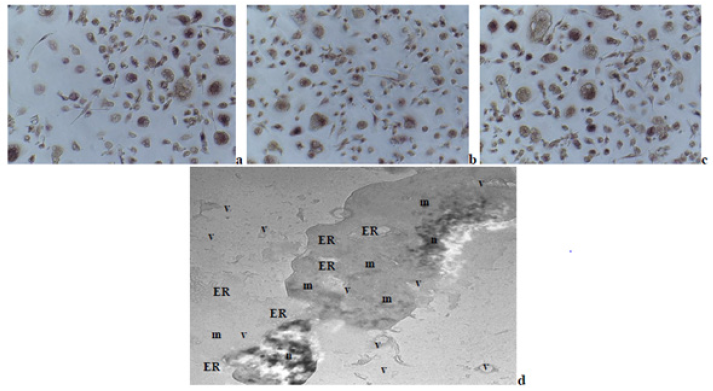
Figure 1: Multi-nuclear osteoclasts, derived from human MSCs, isolated from: a –bone marrow material; b – adipose tissue; c – cartilage tissue (fixed light microscopy preparations, stained by TRAP-technique and Hematoxylin dye, magnification: x100); d – TEM of experimental model, presenting culture of mouse embryonic fibroblasts, pre-cultivated in cultural fluid from previously incubated in it mouse malignant myeloma cells, subsequently freezed in the presence of DMSO and re-incubated. Phase of the fusion process during osteoclast formation could be noted, in which the fusion is between two macrophage-like cells, probably derived because of the presence of malignant cells. In each one of the two cells could be noted a lot of membrane formations, containing vesicles of varying sizes and shapes, including mitochondria, besides in t
On the light microscopy slides could be seen osteoclasts with different sises and shapes, but also in various phases of fusion between myeloid cells, probably in different phases of maturation and differentiation (Figure 1a-1c). All these mature multi-nuclear cells were derived from MSCs of the three different sources, described above. On the electron microscopy slide could be noted a stage of the fusion between two myeloid-like cells, derived from embryonic cellular progenitors (Figure 1d). The two interacting cells are with large sizes, increased cytoplasmic content, and large nucleus with round or kidney shape, respectively, containing several nucleola. These changes could be probably due to the presence of sub-populations of able to differentiate to various directions (depending on the respective conditions) stem-like cells in the general embryonic cell line. Besides mitochondria with different sizes and shapes (round and elongated), also strongly expressed components of ER and vesicle formation could be noted, which proposed increased cellular activity. Additionally, vesicle structures, which include parts of mitochondria and ER besides in the two cells, also in the outside of each one of them, could be noted.
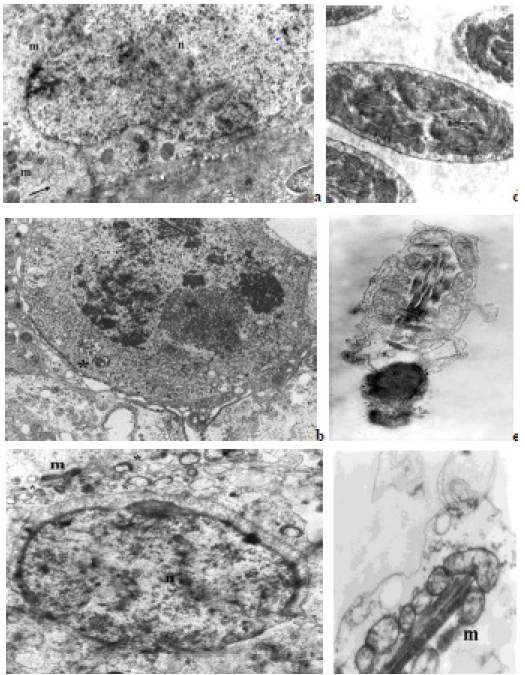
Figure 2: Electron micrographs, showing different mitochondria in germ cells and SCs from human testicular tissue: a - SC with round orthodox type mitochondria (m) and clusters of membrane formations of vesicles (arrow); b - pachytene spermatocyte with two condensed/ intermediate type mitochondria (asterisk); c - round spermatids with condensed type mitochondria (asterisks) around an acrosomal vesicle, as well as condensed (asterisks), and elongated (orthodox) mitochondria (m) in borderline SC; d - segments of forming flagellums with normal mitochondrial sheath and orthodox type mitochondria (cross-sections through the middle piece); e, f – abnormal spermatozoa with defects in the mitochondrial sheath. (n) – nucleus, (m) - orthodox type mitochondria, (*) - condensed/intermediate type mitochondria, (→) - clusters of membrane formations of vesicles, TEM, × 30 000.
These features showed that unlike in the process of derivation of the other types of mature cells from MSCs (osteoblasts/osteocytes, chondrocytes, adipocytes, etc.), in the process of osteoclastogenesis in the mitochondria dynamics besides different intra-cellular cytoskeleton elements, also participate extra-cellular matrix components, which interact with the mitochondrial components. Ultrastructural analysis of histological testicular samples showed a reduced generation of germ cells, principally primary spermatocytes (meiotic cells), round spermatids and Sertoli cells (SCs), (Figure 2a and 2c). Mitochondrial dynamics tracking was performed in four groups of cells: SCs, spermatocytes (meiotic cells), round haploid spermatids, and spermatozoa (Figure 2a-2f). In the SCs, mitochondria are presented mainly by orthodox configuration type (with lamellar cristae), as well as elongated and/or round shapes, dispersed in the cytoplasm. However, although small in number, round mitochondria of intermediate and condensed type are observed located on the periphery of the cells to the border with neighboring germ cells (Figure 2a and 2c). In some of the SCs could be observed clusters of membrane formations containing vesicles of different sizes including mitochondria (Figure 2a). In primary spermatocytes, a small number (2 to 3) of round condensed/ intermediate forms of mitochondria are observed near the nucleus (Figure 2b).
Mitochondria with an elongated shape (resulting from the fusion of these organelles), which are important for maintaining the energy needs of the developing germ cells, are not observed. Round spermatids contain numerous mostly round, condensedtype mitochondria clustered near the forming acrosome (Figure 2c) as well as scattered throughout the cytoplasm. In the concrete case were not observed late (mature) spermatids, were but could be noted as separate fragments of the flagellum (“middle piece”), both with normal and abnormal mitochondrial sheath, and the “principal piece” of parallel developing tails of the male germ cells (Figure 2d). TEM of the ejaculate samples (Figure 1e and 1f) also showed germ cells (spermatozoa) with abnormal morphology. In the “middle piece” of the tail, with normal mitochondrial sheath, the mitochondria have a correct arrangement around the axoneme and orthodox configuration, unlike in the abnormal male gametes (Figure 2e), wherein disorganization and mitochondria of different shapes and sizes are observed (Figure 2f).
Discussion
In the present study, a discussion of mitochondrial dynamics in two aspects of development in two different cell systems is proposed. This is a different research approach to looking for similarities (or differences) in the behavior of mitochondria under different conditions of the cellular environment (including normality and pathology). The results obtained were in agreement with literature data about the possibility of various types of stem/ progenitor cells from different sources to differentiate to various directions by respective appropriate laboratory incubation and external conditions [7,8]. The noted in the embryonic cells from 3T3 cell line could be due to the existence of cellular progenitors in the embryonic cell line, which possess possibilities to differentiate to various directions (including to myeloid direction in the presented study), when appropriate cultivation conditions are available, as the presence of malignant cells/antigens in the concrete case. Furthermore, activated fusion processes could be proposed on the influence of the organic detergent (DMSO) [37], as well as of the drastic temperature changes. Osteoclasts have been proved as rich of mitochondria, which regulate the resorptive activity of these cells by promoting cytoskeletal organization [12].
Other mitochondria-stimulating proteins have been proposed as therapeutic targets in the process of bone resorption. In this way, mediated mitochondrial transfer by autologous MSCs, together with understanding the mechanisms, signal targets and mitochondrial energy metabolism in the stem cells has been suggested as especially important [14]. The results obtained were in agreement with the literature findings [38], but also with our previous data, both in derivation of multi-nuclear osteoclasts from somatic cellular progenitors [39], as well as of mature male germ cells from [40-42]. In this aspect, the mitochondrial dynamics, which has been proved to be influenced mainly by the physiological and stress conditions, could be suggested as underlining the stem cells fate and thus, the respective tissue reparation mechanisms [38]. In this aspect, a relationship of the mitochondrial dynamics with the inflammation has been proposed [43]. On this basis, the age-related chronic systemic inflammation has been associated namely with mitochondrial dysfunction and activated extra-cellular release of mitochondrial components, including fragments of cellfree mtDNA. These data suggest a possibility about development of mitochondrial strategies for application in the stem cell-based regenerative medicine [20]. The observed changes could be explained with the existence of able to differentiate to various directions (including to myeloid direction in the concrete case) cellular progenitors in the embryonic cell line, when appropriate cultivation conditions are available. In this aspect, the mitochondrial dynamics, on the influence of the respective physiological and stress conditions, could be suggested as underlining the stem cells fate [12,14,44]. The proposed interaction of the mitochondreial components besides with intra-cellular, also with extra-cellular components in the processes of fusion was in agreement with the underlining mechanisms, which have been established in a cell-free in vitro-system [45].
The results from the ultrastructural analysis of histological testicular samples (including ejaculate samples) gave additional reason to assume that spermatogenesis is impaired (with probable round spermatid stage arrest) in the case, which could lead to male infertility. The changes in the mitochondrial morphology or dynamics during spermatogenesis are closely dependent on the metabolic and energetic status of cells and have shown a transition from slow to intensive male gamete metabolism [20,42]. According to recent studies, mitochondrial fusion is an essential step in the mitochondrial dynamics during the transition from zygote to pachytene of meiotic cells (in mice), associated with the regulation of increased OXPHOS activity independently of the energy needs in meiosis [20,21]. Another hypothesis about changes in mitochondrial architecture related to osmotic changes in the local cellular tissue environment has also been proposed [2].
In the present study, we can discuss the role of mitochondria dynamics in spermatocytes, spermatids, and spermatozoa, and whether their activity is enough for the energy supply of transformation processes in these cells. Round mitochondria of the condensed/intermediate type (associated with increased OXPHOS activity) are found in pachytene spermatocytes, but they are few, and elongated forms are not observed. The location of mitochondria in a condensed/intermediate form in the proacrosoma zone of the round spermatids is probably connected with the biogenesis and formation of the acrosome. Moreover, in parallel with this process, the structuring of the flagellum of the germ cell begins. In this regard, there is evidence that mitochondrial protein cardiolipin is located in the acrosome, suggesting that besides trans-Golgi vesicles, mitochondria may also be sources of membranes for acrosome biogenesis [5,46]. A reason for the abnormal development of these processes may be a result of impaired mitochondrial dynamics/ plasticity and/or apoptosis. In addition, late/elongated spermatids are not observed. It can be assumed that the clusters of membrane formations, including mitochondria, observed in SC are probably cytoplasmic remnants of reduced (or apoptotic) spermatids, which are subsequently removed as a result of the phagocytic function of Sertoli cells.
It has been shown that Sertoli cells can act as phagocytes and remove apoptotic germ cells and redundant cytoplasm from seminiferous tubules [47,48]. The damages that most often occur in the “middle piece” of spermatozoa were normally associated with disorganization of the mitochondrial sheath (disrupted spiral arrangement of mitochondria) around the axoneme complex, as well as with swollen or obliterated cristae in some the organelles. The observed TEM asymmetry in the mitochondrial size and placement was associated with the disintegration in the structure of the middle piece [38,40]. Often this part of the tail is covered by cytoplasm droplets, containing a multitude of vesicles, ribosomes, and residual nuclear membranes, including mitochondria and other cellular organelles.
This combination of defects in the tails of the morphologically abnormal spermatozoa is most often associated with hypokinesia and/or asthenospermia, and thus, with male infertility. General mechanisms of interaction of extra-cellular matrix components with mitochondrial components in the fusion processes have been proposed in both osteoclast differentiation of MSCs and germ cell maturation [50]. One of the main components of these interactions has been determined by the enzyme DNA synthase and the gene, coding this protein, which is located in the mtDNA. However, the key role of the nuclear genome in the expression of different mtDNA variants in the cell has been characterized. A possibility of the development of new mitochondria-based strategies for various therapeutic needs has been suggested [49].
Conclusion
In the current study was proved the role of the mitochondria in the differentiation of adult human MSCs from several sources to TRAP-positive osteoclasts, depending on the respective incubation conditions applied. In this way, the results obtained show the role of the mitochondria dynamics as a key factor in the cellular differentiation direction. Many properties of the mitochondria are influenced by the physiological conditions and external factors. In this aspect, the activation of mitochondria and their increased amounts in the osteoclasts could be proposed as a protective mechanism against bone resorption by promoting the organization of cytoskeleton components. According to the results of the ultrastructural analysis on male germ cells, the association of impaired and incomplete spermatogenesis with the established abnormalities, such as a reduced number of mitochondria (probably due to altered mitochondrial dynamics) in primary spermatocytes, as well as a lack of elongated/mature spermatids in the germinal epithelium. These data confirmed the view that mitochondrial activity, physiological status, and plasticity are essential for the normal course of spermatogenesis and male fertility. Many features of these organelles are influenced by both physiological conditions and external factors. A possibility about development of new mitochondria-based strategies for the needs of the reparation medicine could also be suggested. Additionally, the current results may contribute to a better understanding of the etiology of male infertility, as well as the possibility of incorporating new mitochondria-based therapies applicable in reproductive medicine and improving in vitro fertilization techniques.
References
- Amaral A, Lourenço B, Marques M, Ramalho Santos J (2013) Mitochondria functionality and sperm quality. Reproduction 146(5): R163-R174.
- Mannella CA (2006) Structure and dynamics of the mitochondrial inner membrane cristae. Biochim Biophys Acta 1763(5-6): 542-548.
- Bereiter Hahn J, Jendrach M (2010) Mitochondrial dynamics. Int Rev Cell Mol Biol 284: 1-65.
- Scott I, Logan DC (2007) Mitochondrial dynamics: the control of mitochondrial shape, size, number, motility, and cellular inheritance. Plant Mitochondria 31: 493-496.
- Ren L, Chen X, Chen X, Li J, Cheng B, et al. (2020) Mitochondrial dynamics: fission and fussion in fate determination of mesenchymal stem cells. Front Cell Dev Biol 8: 580070.
- Mishra P, Chan DC (2016) Metabolic regulation of mitochondrial dynamics. J Cell Biol 212(4): 379-387.
- Seo BJ, Yoon SH, Do JT (2018) Mitochondrial dynamics in stem cells and differentiation. Int J Mol Sci 19(12): 3893.
- Molofsky A, Pardal R, Morrison S (2004) Diverse mechanisms regulate stem cell self-renewal. Curr Opin Cell Biol 16(6):700-707.
- Cloutier A, Ear T, Blais Charron E, Dubois C, McDonald P (2007) Differentiation involvement of NF-κB and MAP kinase pathways in the generation of inflammatory cytokine by human neutrophils. J Leuk Biol 81(2): 567-577.
- Zhang Y, Rohatgi N, Veis DJ, Schilling J, Teitelbaum SL, et al. (2018) PGC1β organizes the osteoclast cytoskeleton by mitochondrial biogenesis and activation. J Bone Miner Res 33(6): 1114-1125.
- Abe T, Sumi K, Kuminatsu R, Oki N, Tsuka Y, et al. (2019) The effect of mesenchymal stem cells on osteoclast precursor cell differentiation. J Oral Sci 61(1): 30-35.
- Xu F, Teitelbaum S (2013) Osteoclasts: new insights. Bone Res 1(1): 11-26.
- Cong Q, Jia H, Li P, Qiu S, Leh J, et al. (2017) P38α MAPK regulates proliferation and differentiation of osteoclast progenitors and bone remodeling in an age-dependent manner. Sci Rep 7: 45964.
- Albensi BC (2019) What is nuclear factor kappa B (NF-κB) doing in and to the mitochondrion? Front Cell Dev Biol 7: 154.
- Zeng R, R Faccio, D Novack (2015) Alternative NF-κB regulates alternative RANKL-induced osteoclast differentiation and mitochondrial biogenesis via independent mechanisms. J Bone Miner Res 30(12): 2287-2299.
- Hayman AR (2008) Tartrate-resistant acid phosphatase (TRAP) and the osteoclast/immune cell dichotomy. Autoimmunity 41(3): 218-223.
- Solberg L, Stang E, Brorson S, Andersson G, Reinholt F (2015) Tartrate-resistant acid phosphatase (TRAP) co-localizes with receptor activator of NF-κB ligand (RANKL) and osteopretegerin (OPG) in lysosomal-associated membrane protein 1 (LAMP1)-positive vesicles in rat osteoblasts and osteocytes. Histochem Cell Biol 143(2): 195-207.
- Yang J, Bi X, Li M (2019) Osteoclast differentiation assay. Methods Molec Biol 1882: 143-148.
- Tilokani L, Nagashima S, Paupe V, Prudent J (2018) Mitochondrial dynamics: overview of molecular mechanisms. Essays Biochem 62(3): 341-360.
- Varuzhanyan G, Chan D (2020) Mitochondrial dynamics during spermatogenesis. J Cell Sci 133(14): jcs235937.
- Zhang Z, Miao J, Wang Y (2022) Mitochondrial regulation in spermatogenesis. Reproduction 163(4): R55-R69.
- Mota P, Amaral S, Martins L, Pereira M, Oliveira P, et al. (2009) Mitochondrial bioenergetics of testicular cells from the domestic cat (felis catus) – a model for endangered species. Reprod Toxicol 27(2): 111-116.
- Pillips B, Gassei K, Orwig K (2010) Spermatogonial stem cell regulation and spermatogenesis. Philos Trans R Soc Lond B Biol Sci 365(1546): 1663-1678.
- Ramalho Santos J, Varum S, Amaral S, Mota P, Sousa A, et al. (2009) Mitochondrial functionality in reproduction: from gonads and gametes to embryos and embryonic stem cells. Hum Reprod Update 15(5): 553-572.
- Zvetkova E, Ilieva I, Sainova I, Nikolov B (2018) Sperm mitochondrial biology during spermatogenesis and fertilization. Acta morphologica et anthropologica 25(3-4): 171-181.
- Meinhardt A, Wilhelm B, Seitz J (1999) New aspects of spermatogenesis. Expression of mitochondrial marker proteins during spermatogenesis. Hum Reprod Update 5(2): 108-119.
- Hackenbrock C (1968) Ultrastructural bases for metabolically linked mechanical activity in mitochondria. II. Electron transport-linked ultrastructural transformations in mitochondria. J Cell Biol 37(2): 345-369.
- Storey B (2008) Mammalian sperm metabolism: oxygen and sugar, friend and foe. Int J Dev Biol 52(5-6): 427-437.
- Miki K, Qu W, Goulding E, Willis W, Bunch D, et al. (2004) Glyceraldehyde 3-phosphate dehydrogenase-S, a sperm-specific glycolytic enzyme, is required for sperm motility and male fertility. Proc Natl Acad Sci USA 101(47): 16501-16506.
- Mukai C, Okuno M (2004) Glycolysis plays a major role for adenosine triphosphate supplementation in mouse sperm flagellar movement. Biol Reprod 71(2): 540-547.
- Nakamura M, Okinaga S, Arai K (1984) Metabolism of pachytene primary spermatocytes from rat testes: pyruvate maintenance of adenosine triphosphate level. Biol Reprod 30(5): 1187-1197.
- Yan W, Diao S, Fan Z (2021) The role and mechanism of mitochondrial functions and energy metabolism in the function regulation of the mesenchymal stem cells. Stem Cells Res Ther 12(1): 140.
- Strober G (2015) Trypan blue exclusion test of cell viability. Curr Protoc Immunol 2(111): A3.B.1-A3.B.3.
- Adolfsson J, Mansson R, Buza Vidas N, Hultquist A, Liuba K, et al. (2005) Identification of Flt3+ lympho-myeloid stem cells lacking erythro-megakaryocytic potential a revised road map for adult blood lineage commitment. Cell 121(2): 295-306.
- Susa M, Luong Nguyen N, Cappelen D, Zamurovic N, Gamse R (2004) Human primary osteoclasts: in vitro generation and applications as pharmacological and clinical assay. J Translat Med 2(1): 6.
- Kadir RA, Ariffin SHZ, Wabat RMA, Kermani S, Senafi S (2012) Characteriztion of mononucleated human peripheral blood cells. Sci World J.
- Norwood TH, Ceigler CJ, Martin GM (1976) Dimethyl sulfoxide enchances polyethylene glycolmediated somatic cell fusion. Somat Cell Genet 2(3): 263-270.
- Folgero T, Bertheussen K, Lindal S, Torbergsen T, Oian P (1993) Mitochondrial disease and reduced sperm motility. Hum Reprod 8(11): 1863-1868.
- Sainova I, Ilieva I, Valkova I, Nikolov B, Nikolova E (2015) Development of different microscopic methods for detection of cell changes and intra-cellular interactions. J BioSci Biotechnol: Special Edition from National Youth Conference “Biological Sciences for Better Future”, 30-31 October, Plovdiv, Bulgaria, 9-14.
- Ilieva I, Ivanova S, Tzvetkova P, Nikolov B (2011) Electron microscopic studies of abnormal sperm in the pathology of the male reproductive system. Compt rend Acad bulg Sci 64(3): 275-382.
- Ilieva I, Tzvetkova P, Sainova I, Taushanova P, Kacarov M, et al. (2016) Immature spermatogenic cells in semen fluids of infertile men. Compt Rend Acad Bulg Sci 69(1): 85-94.
- Ilieva I, Sainova I, Zvetkova E (2017) Sperm mitochondria-associated male infertility: sperm quality defects and mitochondria (mtDNA) anomalies. Acta morphologica et anthropologica 24(3-4): 114-122.
- Picca A, Guerra F, Calvani R, Romano R, Coelho Junior HJ, et al. (2022) Circulating mitochondrial DNA and inter-organelle contact in ageing and associated conditions. Cells 11(4): 675.
- Goldberg E, Eddy E, Duan C, Odet F (2010) LDHC: the ultimate testis-specific gene. J Androl 31(1): 86-94.
- Schauss AC, Huang S, Choi SY, Xu L, Soubeyrand S, et al. (2010) A novel cell-free mitochondrial fusion assay amenable for high-throughoutput screenings of fusion modulators. BMC Biology 8: 100.
- Khawar MB, Gao H, Li W (2019) Mechanisms of acrosome biogenesis in mammals. Front Cell Dev Biol 7: 195.
- Nicholls PK, Stanton PG, Chen JL, Olcorn JS, Haverfield JT, et al. (2012) Activin signaling regulates Sertoli cell differentiation and function. Endocrinology 153(12): 6065–6077.
- Sharpe RM, McKinnell C, Kivlin C, Fisher JS (2003) Proliferation and functional maturation of Sertoli cells, and their relevance to disorders of testis function in adulthood. Reproduction 125(6): 769–784.
- Mohammadalipour A, Dumbali SP, Wenzel PL (2020) Mitochondrial transfer and regulators of mesenchymal stromal function and therapeutic efficacy. Front Cell Dev Biol 8: 603292.
- Knorre DA (2019) Intracellular quality control of mitochondrial DNA: evidence and limitations. Philos Trans R Soc Lond B Biol Sci 375(1790):20190176.