Research Article
Creative Commons, CC-BY
On the Influence of Sports Specialization on the Work of Muscle-Tendon Mechanics in Highly Qualified Athletes
*Corresponding author: Mikhail Shestakov, Federal Science Center of Physical Culture and Sport, Moscow, Russia.
Received: November 01, 2023; Published: November 06, 2023
DOI: 10.34297/AJBSR.2023.20.002724
Abstract
The article discusses the research findings on jumping strategies used by elite athletes in different sports. The hypothesis states that athletes employ different movement patterns and different features of Muscle-Tendon Unit (MTU) functions when performing the same task. The selection of a muscle contraction mechanism depends on the sport discipline and the unique characteristics of the movement.
The study analysed Russian athletes from various national teams in alpine skiing and mogul. They performed drop jumps from heights of 0.1, 0.3, and 0.5m without using their arms for swinging. Data was collected using the Qualisys motion capture system (400 frames per second) and two force plates, the AMTI 6000. The authors performed calculations to determine the accumulation and utilization of elastic strain energy and assessed the metabolic energy expended in the Muscle-Tendon Unit (MTU). They concluded that the varied strategies observed in drop jumps can be attributed to the transfer of sport-specific motor skills. These findings can potentially assist coaches in designing personalized training plans for athletes, including targeted strength exercises for specific muscle groups.
Keywords: Muscle-tendon unit, Drop-jump, Elite athlete, Elastic energy
Introduction
Elastic strain energy exists in tendons and plays a crucial role in different motor tasks. Its primary function is to reduce the energy expenditure required for muscle contractions and enhance the power output of Muscle-Tendon Units (MTUs) [1]. The importance of this concept lies in optimizing performance in various sports movements. By aligning the functioning of MTUs with the specific demands and characteristics of a particular sport discipline, athletes can improve their results.
Based on the hypothesis [2], it is suggested that when performing a motor task, a muscle must generate sufficient power to meet the mechanical demands of the task and initiate movement. The timing, sequence, and magnitude of muscle activation are crucial factors in determining the specific movement.
In the case of multi-joint movements in the lower extremities during take-off, two elastic mechanisms contribute to the efficiency of power generation. These mechanisms include the pre-stretch of skeletal muscles and the transfer of mechanical energy through biarticular muscles.
The utilization of each of these mechanisms is believed to have an impact on the overall strategy employed for organizing multi- joint movements. One of the primary mechanisms that enhances the power of a take-off is the preliminary stretching of skeletal muscles. This mechanism is well-known for its ability to increase the strength of subsequent Muscle-Tendon Complex (MTU) contractions [3,4]. It is observed in various movements, such as vertical jumps [5].
The second mechanism, known as mechanical energy transfer, also contributes to the augmentation of power during a take-off. This mechanism has been studied in relation to activities like running, hopping, and vertical jumping [3-6]. The transfer of mechanical energy via biarticular muscles is closely associated with the effects experienced in the MTU [7].
Our hypothesis posits that athletes may employ different movement organization strategies to accomplish the same motor task. The selection of a particular mechanism to enhance muscle contraction power is influenced by the specific characteristics of the sport discipline, particularly the demands placed on the primary exercise.
The findings from this study will be valuable for coaches in developing individualized training plans for athletes. Specifically, these results can inform the design of strength training exercises that target specific muscle groups, based on the identified movement organization strategies.
Methods
The study involved male members of the Russian national teams in alpine skiing n=4 (weight 82,4±4,3 kg; height 1,79±3,1 m; experience 10,1±3,1 years) and mogul skiing n=5 (weight 73,5±2,3 kg; height 1,66±2,4 m; experience 7,8±1,8 years). All athletes took part in the World Cups and World Championships. The experiment was carried out within the framework of regular testing of national team members according to established protocols in the course of preparation for international competitions [8].
Testing procedure. Following a warm-up session, the participants engaged in drop jumps, which involved vertical jumps from heights of 10cm, 30cm, and 50cm without utilizing arm swings. The subjects were instructed to wear their preferred athletic shoes and to position their hands on their hips during the jumps. The trial with the highest jumping height, representing the elevation of the Centre of Mass (CoM), was selected as the best out of three attempts and used for subsequent analysis. The rest period between trials ranged from approximately 2-3 minutes, depending on the individual requirements of each athlete.
Data Processing Approach. The Qualisys Motion Capture System, consisting of 24 cameras (Oqus 5, Qualisys, Sweden), was used to collect real-time movement data. The jumping exercises were performed on two AMTI 6000 force plates (AMTI, USA). The recording was conducted at a frame rate of 400 frames per second and synchronized with the signals from the force plates. The collected data was processed using the Open Sim software package [9]. This software package facilitated the creation of individualized musculoskeletal models for each athlete, allowing for the identification of unique characteristics in their movement technique. To perform kinematic and dynamic calculations, we utilized a full-body model based on the work of Hamner and Delp [10]. The model employed was a three-dimensional musculoskeletal model with 29 degrees of freedom, featuring torque actuators that drove 92 muscles in the torso and lower extremities.
This model has been previously utilized to investigate the contribution of individual muscles to the acceleration of the body’s centre of mass during a jump [10,11]. In our study, we focused on five of the 35 lower limb muscles included in the model. To analyse the metabolic costs associated with the jump experiment, we selected a specific group of key muscles involved in the take-off phase of a vertical jump. These muscles include: Gl (gluteus maximus, gluteus Medius, and gluteus minimums), RF (Rectus Femoris), VAS (Vastus Medialis), GAS (lateral sections of the gastrocnemius), and SOL (Soleus).
The individual muscle and tendon complex was represented using a three-piece Muscle-Tendon Unit (MTU) model, which was based on Thelen’s work in 2003 [12]. This model was implemented in the Open Sim application and allowed for the calculation of muscle and tendon length changes and strength variations across a wide range of body positions. The MTU model provided a detailed analysis of the functioning of the ankle, knee, and hip joint MTUs in generating force and its derivatives for each subject. The simulation of each jump was conducted using the methods described by Hamner and Delp [10]. Our simulation workflow commenced with scaling the geometry of the generic musculoskeletal model to fit the anthropometry of each subject. This scaling process was accomplished using the Open Sim Scale Tool. Additionally, we scaled the maximum isometric forces of the muscles based on regression equations that considered the subject’s height and mass [11].
Next, we generated muscle-driven motions for the recorded experiments using Open Sim’s Computed Muscle Control (CMC) Tool [12]. This involved utilizing the individualized models and adjusted kinematics. The CMC Tool calculated muscle excitations that could produce the observed jumping motion while minimizing the sum of squared muscle activations at regular intervals throughout the motion.
In our analysis, we aimed to estimate the amount of Elastic Strain Energy (ESE) that could be stored and utilized. To accomplish this, we employed methods proposed by researchers in previous studies [3,13]. To estimate metabolic energy consumption, we utilized a metabolic model developed by researchers [14]. For this purpose, we used the Umberger2010MuscleMetabolicsProbe within Open Sim v4.
Following the calculation method, we summed the rate of energy expenditures for all muscles and added a basal rate of 1.2W/ kg [15] to determine the overall metabolic energy expended. For analysing the results of our research, we utilized the STATISTICA software package, specifically version 10. Due to the relatively small sample sizes, we employed non-parametric statistical methods, namely the Kruskal-Walli’s test and the Wilcoxon test. These tests are suitable for analysing data that may not meet the assumptions of parametric statistical tests.
Ethical Approval. The study underwent approval by the Local Human Research Ethics Committee, and prior to participating, all participants provided their written informed consent. The human testing procedures adhered to the principles outlined in the Declaration of Helsinki.
Results
Figure 1 demonstrates multidirectional changes in the maximum peak power in drop jumps from different heights performed by the subjects. Mogul skiers showed negative dynamics of the maximum peak power as the height of the drop jump increased: the maximum peak power in drop jumps from the height of 0.1m and 0.3m differed by 2.67±0.05 W/kg ((T=0,0, p=0,043 ), the difference of the maximum peak power in drop jumps from the height of 0.3 m and 0.5 m was 2.89±0.07 W/kg (T=0,0 p=0,041). The alpine skiers showed positive dynamics of maximum peak power.
Mogul skiers demonstrated significant difference in maximum peak power in comparison with the alpine skiers (H= 8,22003 p<0,01) (Figures 1,2).
If we compare Figures 1 and 2, we will note that the athletes achieved different power of movement on the background of different leg stiffness. The dynamics of data within each group was different. Mogul skiers demonstrated the maximum values in leg stiffness in drop jumps from 0.5m. In alpine skiers the maximal leg stiffness was found in drop jumps from 0.1m. The small height may permit these athletes to perform preliminary muscle stimulation before landing that makes it possible to counteract external inertial forces acting on the body. When the height is greater, athletes try to compensate loads on the musculoskeletal system in shock absorption phase by increasing the amplitude of movement in the knee joints, thereby reducing the stiffness of the legs (Figure 3).
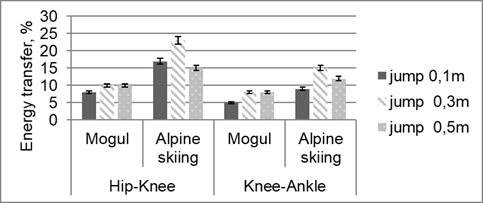
Figure 3: Transfer of energy between muscle groups of the lower limbs in the take-off phase (concentric muscle contraction).

Note*: Gl-gluteus maximus, gluteus medius, gluteus minimus muscles, RF-rectus femoris, VAS-vastus medialis, GAS-lateral sections of the gastrocnemius muscle, SOL-soleus muscle.
Figure 4: Metabolic expenditures in simulated muscles.
Energy transfer between the muscle groups of the lower extremities during the concentric contraction phase of the take-off was calculated using the method described in [4] (Figure 3). The highest percentage of energy transfer was found in alpine skiers (Hip-Knee: 23±0.4% from 0.3м), but the segments of the lower limbs mostly involved in the energy transfer were different in those two groups. In alpine skiers’ energy transfer between the hip extensors and the thigh muscles was the highest. Mogul skiers showed a very low energy transfer from the hip extensors to the thigh muscles and almost no energy transfer from the thigh muscles to the lower leg muscles via the knee joint (Figure 4).
The highest peak metabolic costs of all lower extremity’s muscles were found in mogul skiers (Figure 4). In all athletes peak metabolic costs of the hip extensor (Gl) tended to decrease, as the height of drop jumps increased, because athletes tried to maintain more upright posture for stability. All athletes had the lowest peak metabolic costs in GAS. High peak metabolic costs in RF and VAS were found in all athletes (87.2-73.1 and 105.1-74.6 W, correspondingly). As the height of drop jumps increased, the metabolic costs in RF and VAS_L increased in mogul skiers and decreased in alpine skiers. The total metabolic costs increased in mogul skiers, were stable in alpine skiers, when the height of drop jumps increased (Figure 5).
The peak force in the tendon of the MTU model involved in the take-off is shown in Figure 5. Alpine skiers and mogul skiers demonstrated active work in the tendons of the GAS and SOL muscles, as well as positive dynamics, as the height of drop jumps increased. Tendon activity of VAS in mogul skiers and alpine skiers revealed similarity in magnitude and positive dynamics. The greatest peak activity of the Gl tendons during the take-off was observed in alpine skiers when drop jumping from a height of 0.5 m (108.3±6.7N).
Discussion
In order to comprehend the unique characteristics of Muscle- Tendon Unit (MTU) function in athletes from distinct sport specializations, it is essential to provide a brief overview of the specific features of each sport discipline.
Alpine Skiing
Alpine skiing consists of four disciplines: Slalom (SL), Giant Slalom (GS), Super Giant (SG), and Downhill (DH). In slalom, the peak Ground Reaction Force (GRF), which measures the external load on the skier and equipment, can reach up to five times the athlete’s body weight [16]. The highest GRF values in the other alpine skiing disciplines are observed in giant slalom [17]. The key muscle groups involved in alpine skiing include the thigh muscles, hamstrings, quads, calves, and foot muscles [18]. These muscles predominantly engage in eccentric contractions during the race, where the muscle lengthens during contraction. The contraction rate is relatively slow in downhill skiing compared to activities like running or jumping due to the minimal changes in hip angle during a ski turn. Alpine skiers exhibit high levels of isometric and isokinetic leg strength compared to other athletes [19]. In technical disciplines, a “quasi-static” component of skiing has been proposed, highlighting evidence of coactivation during thigh muscle contractions [20]. Research on elite Swiss riders has confirmed that eccentric muscular work prevails over concentric work in slalom, giant slalom, and super giant disciplines [21]. This suggests that alpine skiing is unique among sports for its emphasis on eccentric muscular activity [21]. The higher force levels in alpine skiing allow skiers to achieve greater control in turns and experience less fatigue during races.
Mogul Skiing
Mogul skiing involves navigating through irregularities such as bumps and pits while descending a mountain. On a mogul course, turns are made cyclically to the left and right without a separate transition phase between individual turns. Skiers’ maneuverer around the bumps, creating a “swinging rhythm”. This type of skiing is characterized by distinct phases of ski loading and unloading, as well as ski bending and unbending.
During mogul skiing, the load is distributed nearly evenly between both skis, with each ski experiencing a maximum load of approximately 150% of the skier’s body weight. Athletes must maintain knee joint angles close to 90°, ensuring minimal deviation of the trajectory of the Centre of Mass (CoM). This stance enables skiers to absorb height differences in bumps and pits while managing and controlling their speed. Research has shown that in mogul skiers, as opposed to alpine skiers, the ratio of eccentric to concentric patterns closely resembles that seen in running [22].
The results presented in the findings indicate that athletes from different groups employed distinct strategies to generate muscle power and achieve maximum jump height in drop jumps. Our aim is to elucidate the mechanisms underlying the functioning of lower extremity muscles in athletes who exhibit varying movement organization and muscle-tendon interaction during drop jumps. Specifically, we will explore approaches that enhance Muscle-Tendon Unit (MTU) contraction by leveraging the elastic properties of muscles, particularly their resilient elements, which lead to muscle potentiation. It is widely acknowledged in the literature that pre-stretching the MTU enhances subsequent contraction, a concept supported by numerous authors. However, studying the behaviour of the human MTU in vivo, particularly during complex multi-joint movements, poses challenges. While we do not delve into the mechanisms of MTU pre-stretching, we will examine whether the athletes in our study benefited from the positive effects of MTU pre-stretch. The duration of take-off in both alpine skiers and mogul skiers extended beyond the time frame suitable for utilizing the stretch reflex. However, these athletes were still able to enhance voluntary muscle contraction through the elastic strain energy, despite the extended shock absorption phase. Although an increase in the time for Muscle- Tendon Unit (MTU) stretching reduces the efficiency of elastic strain energy utilization, this mechanism remains intact [23].
We may find it perplexing because the optimal ratio between muscle length and the speed of its stretching is disrupted in alpine and mogul skiers due to the large angular amplitudes of movements in leg joints. Nevertheless, the use of elastic energy remains possible regardless of muscle length [24]. This raises the question of how the positive effect of shock absorption is utilized in drop jumps performed by alpine and mogul skiers. Clearly, the MTU work differs in these athlete groups due to the different techniques involved in their sport disciplines.
This distinction is supported by the data concerning the transfer of elastic deformation energy among the lower extremity joints. Specifically, let’s examine the results of a drop jump from a height of 0.3 meters, where the load on the leg muscles is optimal for achieving maximum jump height [25]. In this jump, the potential use of elastic energy during the take-off phase was approximately 23±1.6% (Hip-Knee) and 15±1.1% (Knee-Ankle) in alpine skiers, and 12±0.6% (Hip-Knee) and 8±0.5% (Knee-Ankle) in mogul skiers. Alpine skiers demonstrated more effective energy transformation due to the higher stiffness of their leg muscles. The difference in the utilization of stored elastic energy between the two groups was evident in comparisons of metabolic costs in Rectus Femoris (RF) and vastus lateralis (VAS) muscles, as well as in tendon strength, which were significantly higher in mogul skiers.
In a drop jump from a height of 0.5meters, the external load on the leg muscles was almost critically high. Mogul skiers experienced a decrease in mechanical power and an increase in metabolic costs and tendon strength in the RF muscle and VAS_L muscle. Both groups showed a decrease in the utilization of elastic energy. Considering the varying demands of each sport discipline, we can assume that mogul skiers do not face mandatory requirements for generating maximum power during a mogul race, unlike alpine skiers. In alpine skiers, the decrease in energy transfer efficiency may be associated with a decrease in stiffness in order to increase the role of muscle activity.
Conclusion
The findings demonstrated the significant impact of sport specialization on motor control in elite athletes. By analysing drop jumps performed at varying heights, we uncovered patterns in the functioning of the Musculo Tendinous Unit (MTU) that resembled those observed during competitive exercises. This enabled us to gain valuable insights into the various mechanisms that contribute to generating movement power in top athletes across different sports.
In conclusion, continuing research in assessing the effectiveness of elite athletes should involve an individualized approach that considers the unique aspects of each athlete’s sport technique. Exploring conscious control of muscles and the body, along with regularities related to generating power in movements and the behaviour of MTUs, can lead to valuable insights for coaches. These insights can aid in selecting appropriate training methods, developing specialized exercises, and designing effective training regimens in different sport disciplines.
Conflict of Interest
The authors declare no conflict of interest.
Acknowledgement
None.
References
- Lichtwark GA, Wilson AM (2005) Effects of series elasticity and activation conditions on muscle power output and efficiency. J Exp Bio 208(15): 2845-2853.
- Bobbert MF, Ingen Schenau GJ (1988) Coordination in vertical jumping. J Biomech 21(3): 249-262.
- Prilutsky BI, Zatsiorsky VM (1994) Tendon action of two-joint muscles: transfer of mechanical energy between joints during jumping, landing, and running. J Biomech 27(1): 25-34.
- Prilutsky BI, Petrova LN, Raitsin LM (1996) Comparison of mechanical energy expenditure of joint moments and muscle forces during human locomotion. J Biomech 29(4): 405-415.
- Stafilidis S, Tilp M (2015) Effects of short duration static stretching on jump performance, maximum voluntary contraction, and various mechanical and morphological parameters of the muscle-tendon unit of the lower extremities. Eur J Appl Physiol 115(3): 607-617.
- Bobbert M, Zandwijk JV (1994) Dependence of human maximum jump height on moment arms of the bi-articular m. gastrocnemius; a simulation study. Human Movement Science 13: 697-716.
- Lai A, Schache AG, Lin YC, Pandy MG (2014) Tendon elastic strain energy in the human ankle plantar-flexors and its role with increased running speed. J Exp Biol 217(17): 3159-3168.
- Shestakov M (2015) Use of motion capture system in Russian teams training for Sochi Olympic Games. Malmö
- Delp SL, Anderson FC, Arnold AS, Loan P, Habib A, et al. (2007) Open Sim: open-source software to create and analyze dynamic simulations of movement. IEEE Trans Biomed Eng 54(11): 1940-1950.
- Hamner SR, Delp SL (2013) Muscle contributions to fore-aft and vertical body mass center accelerations over a range of running speeds. J Biomech 46(4): 780-787.
- Hamner SR, Seth A, Delp SL (2010) Muscle contributions to propulsion and support during running. J Biomech 43(14): 2709-2716.
- Thelen DG, Anderson FC, Delp SL (2003) Generating dynamic simulations of movement using computed muscle control. J Biomech 36(3): 321-328.
- Delp SL, Loan JP (1995) A graphics-based software system to develop and analyze models of musculoskeletal structures. Comput Biol Med 25(1): 21-34.
- Umberger BR, Gerritsen KGM, Martin PE (2003) A model of human muscle energy expenditure. Comput Methods Biomech Biomed Engin 6(2): 99-111.
- Uchida TK, Hicks JL, Dembia CL, Delp SL (2016) Stretching Your Energetic Budget: How Tendon Compliance Affects the Metabolic Cost of Running. PLoS One 11(3): e0150378.
- Supej M, Kugovnik O, Nemec B (2002) New advances in racing slalom technique. Kinesiologia Slovenica 8: 25-29.
- Gilgien M, Spörri J, Kröll J, Crivelli P, Müller E (2014) Mechanics of turning and jumping and skier speed are associated with injury risk in men’s world cup alpine skiing: a comparison between the competition disciplines. Br J Sports Med 48(9): 742-747.
- Gilgien, M, Kröll, J, Spörri, J, Crivelli P, Müller E (2018) Application of dGNSS in alpine ski racing: basis for evaluating physical demands and safety. Front Physiol 9: 145.
- Astrand PO, Rodahl K. Textbook of work physiology: Physiological bases of exercise 3rd
- Hintermeister RA, O’Connor DD, Dillman CJ, Suplizio CL, Lange GW, et al. (1995) Muscle activity in slalom and giant slalom skiing. Med Sci Sports Exerc. 27(3): 315-322.
- Voigt M, Simonsen EB, Dyhre Poulsen P, Klausen K (1995) Mechanical and muscular factors influencing the performance in maximal vertical jumping after different prestretch loads. J Biomech 28(3): 293-307.
- Tesch PA (1995) Aspects on muscle properties and use in competitive Alpine skiing. Med Sci Sports Exerc 27(3): 310-314.
- Aruin AS, Zatsiorsky VM (1984) Biomechanical characteristics of human ankle-joint muscles. Eur J Appl Physiol Occup Physiol 52(4): 400-406.
- Shalmanov AA (2002) Methodological foundations of studying motor actions in sports biomechanics. Moscow 48.
- Vogt M, Hoppeler H (2016) The role of eccentric exercise training in alpine ski racers. In Eccentric Exercise, Physiology and Application in Sport and Rehabilitation pp.132-147.