Review Article
Creative Commons, CC-BY
Contribution of Simulation Method to Estimation of Pediatric Radiation Dose for Abdominal and Pelvic Computed Tomography Procedures: Systematic Review
*Corresponding author:Khalid M Aloufi, Department of Diagnostic Radiology Technology, College of Applied Medical Sciences, Taibah University, Al Madinah, Kingdom of Saudi Arabia.
Received: January 19, 2024; Published: January 30, 2024
DOI: 10.34297/AJBSR.2024.21.002836
Abstract
Introduction: It is essential to assess accurately the radiation dose from different radiological procedures, especially for pediatric patients. This is because pediatric patients are more radiosensitive than adults and their life expectancy is longer. CT is considered as one of the highest radiation dose sources and is common among medical imaging modalities. Simulation method (SM) is a powerful tool to assess radiation dose for CT procedures and can overcome the limitations of real measurements.
Aim: This paper aims to provide a systematic review of the contribution of SM to estimation of pediatric radiation dose for abdominal and pelvic CT procedures.
Methodology: A wide internet database search was conducted, covering the period from January 1990 to September 2023 to find published papers in SM to estimate pediatric radiation dose for abdominal and pelvic CT procedures. Pediatric age range was assumed to be from birth to 16 years old. The database search was performed via PubMed/Medline and other databases. 31 published papers matched the inclusion criteria of this study, whereas 1,944 papers were excluded.
Results: The highest frequency of publication was during 2015 (39%). Domains of the reviewed studies were classified according to (1) purpose, (2) methodology, and (3) finding. The purposes among the included published papers were distributed approximately equally. Using Simulation codes and voxelized phantoms were dominant methodology with 41.9% and 77.4% respectively. SM estimation of equivalent dose dominated the reviewed studies with 81%. 74.2% of the included studies reported that their results were strongly related to the characteristics of simulated CT scanner or simulated phantom. A few SM studies examined tissue weighting factors and conversion factors.
Conclusions: SM results with the same conditions are comparable. Using CT dose calculators will be the dominant method for assessing CT dose in the near future. Even though Adequate studies were found, conducting studies for specific subjects are recommended. Recommendations: More studies are recommended to investigate SM results when applying different variables, such as tissue weighting factors and conversion factors. Additional studies are recommended to compare the results from different CT dose calculators.
Keywords: Simulation, Computed Tomography, Radiation Dose, Pediatric, Abdomen, Pelvis
Introduction
Pediatric patients are more radiosensitive than adults and their life expectancy is longer. Thus, it is essential to assess radiation dose from different radiological procedures accurately. This is to optimize radiological procedure, to minimize radiation dose and then, to reduce potential risks associated with ionizing radiation [1-3]. Priority is given to assessing radiation dose in cases of the highest dosage and the most common radiological procedures.
Computed Tomography (CT) is considered as one of the highest radiation dose sources and one of the most common among medical imaging modalities [4]. Simulation Method (SM) is computational method used to predict the results from statistical trails [5]. SM is a powerful tool for assessing radiation doses for CT procedures. This is because SM can be used for radiation dose estimation in complicated human body tissues and organ structures, whereas it is impossible to conduct real experimental procedures [6,7]. In addition, SM can accurately estimate the risks associated with these radiation doses. Factors affecting radiation dose, such as patient size, age, and exposure, can be examined using SM. Radiation dose estimation for CT examinations can be performed using SM based on CT dose calculators or codes.
Using CT dose calculators is a straightforward and simple method of estimating radiation dose. Several CT dose calculators are available: CT-Expo, DoseWatch ImPACT, ImpactDose, NCICT, VirtualDose CT, Radimetrics and WinDose. Some of them are commercials and a few are free. There are some differences between the properties of these CT dose calculators, such as the properties of used computational phantom, tube current modulation modeling and user interface platforms. However, they are comparable in accuracy and results [8-10]. Simulation codes are simulation of radiation physics, geometry and tracking radiation interactions thought the geometry [11]. Examples of these codes are Geometry and Tracking 4 (Geant4) code [12], MC N-Particle (MCNP) code [13], FLUktuierende KAskade (FLUKA) code [14] and Electron Gamma Shower (EGS) code [15]. These codes can be used (1) to assess radiation dose, (2) to assess image quality, (3) to analyze x-ray tube outputs (4) to optimize x-ray room designs and (5) to simulate pathology images [16-20].
To validate the accuracy of certain SM results, comparisons SM results, real measurements results and results from published studies were performed [9,21,22]. Simulation codes are more complicated compared with CT dose calculators, because of the complications in CT scanner and computational human body phantom descriptions. This paper aims to provide a systematic review of the contribution of SM to estimation of pediatric radiation dose for abdominal and pelvic CT procedures.
Methodology
Literature Search and Inclusion Criteria
A wide search of internet databases was conducted for the period from January 1990 to September 2023 to find papers published in the “contribution of SM to pediatric radiation dose estimation for abdominal and pelvic CT procedures”. In this systematic review, pediatric age range was assumed to be from birth to 16 years old. The database search was performed via PubMed/Medline and other databases; for example, ScienceDirect, Semantic Scholar and Google Scholar. The keywords used in this database search were “Simulation, radiation dose, pediatric, and abdominal pelvic computed tomography ([Simulation or Monte Carlo] and [pediatric or pediatrics] and radiation dose AND [computed tomography or CT])”. In the internet databases search, the method selected for estimating the pediatric radiation dose for abdominal and pelvic CT procedures was the SM using codes and CT dose calculators.
The inclusions were published papers in SM of radiation dose estimation for abdominal and pelvic CT procedures of pediatric patients (i.e., from birth to 16 years old). The exclusions were published papers in SM of other CT procedures, such as brain, chest and extremities, as well as CT procedures for young and adult patients (i.e., over 16 years old). In addition, radiotherapy and other diagnostic procedures rather than CT scan using ionizing or nonionizing methods or radioactive materials were excluded, for instance, general radiography, fluoroscopy, interventional radiology, ultrasound, magnetic resonance and nuclear medicine. The search strategy used to identify the publications for this systematic review is shown in (Figure 1).
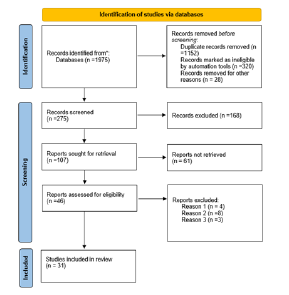
Figure 1: Search strategy used to identify articles for the systemic review regarding PRISMA guidelines. The search was on PubMed/ MEDLINE, ScienceDirect, Semantic Scholar, and Google Scholar. Some records were excluded because they were reviews, reports, dissertations, seminar papers, recommendations, and others. Reason 1: articles were removed from the websites. Reason 2: articles were not available in English language. Reason 3: articles were not accessible.
Then, papers published on PubMed/Medline and other database websites on SM of pediatric radiation dose for abdominal and pelvic CT procedures were reviewed. 1,975 papers published between January 1990 and September 2023 were reviewed regarding the keywords, the papers’ titles, their abstracts, aims, methodologies and findings. 31 published papers matched the inclusion criteria of this study, whereas 1,944 papers were excluded. The majority of the excluded published papers were for adult patients’ dosimetry in diagnostic and radiotherapy fields. In addition, others were concerned with therapeutic beam line simulation, optimization of radiation dose delivery in radiotherapy. Nevertheless, some related published papers might be missing.
Quality Assessment of Selected Published Papers
The criteria of the published papers were selected to be eligible, and then included in this systematic review. Duplicated published papers were found and then removed. Published papers were selected to include publication information (authors and published year), purpose, methodology and finding.
Ethics Statement
Ethical approval was waived for this study, because there were no human participants, and the study was based on website searching.
Results
The distribution (over 1990 to 2023: 33 years), of the included published papers in this systematic review is illustrated in Figure 2, and the used SM (i.e., CT dose calculators and simulation codes) over 1990-2023 (33 years) is shown in Figure 3.

Figure 2: Distribution, over 1990 to 2023 (33 years), of the included published papers in this systematic review.
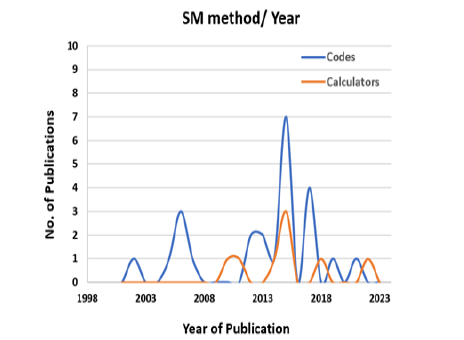
Figure 3: Distribution of used SM (i.e., CT dose calculators or simulation codes) over 1990-2023 (33 years).
In addition, the results comparisons for simulated organ absorbed dose (mGy) and simulated effective dose (mSv) from several studies are shown in Table 1.
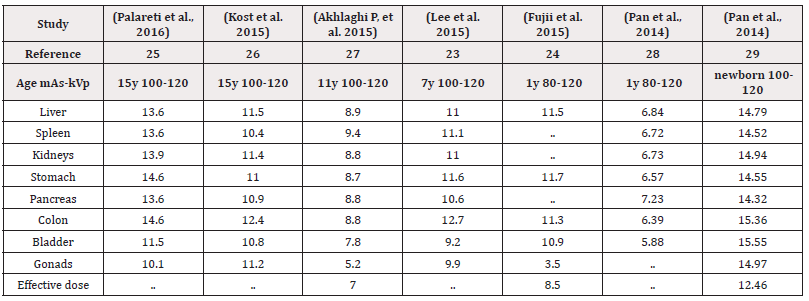
Table 1: Comparisons of simulated organ absorbed dose (mGy) and simulated effective dose (mSv) from several studies.
Domains
Domains of the reviewed studies were classified according to (1) purpose, (2) methodology, and (3) finding. The classification was D.(1) for purposes, D.(2) for methodology and D.(3) for finding. Then, each domain was subdivided into subdomains. Subdomains classification was D. (1.1), D.(1.2), D.(1.3), ... for purposes, D.(2.1), D.(2.2), D.(2.3), ... for methodology and D.(3.1), D.(3.2), D.(3.3), ... for finding. The subdomains are chosen according to the frequent subjects included in the published papers. Then, the included published papers were distributed and sorted regarding the frequent subjects of the subdomains, as shown in Table 2.

Table 2: The numbers of published studies in frequent subject of the subdomains for purpose, methodology and finding.
Domian D.(1): (1) is the domain of purpose for the included published papers in this systematic review. D.(1) includes seven subdomains. The Subdomains are D. (1.1), D. (1.2), D.(1.3), D.(1.4), D.(1.5), D.(1.6), and D.(1.7). Selection of the subdomains subjects was regarding frequent purposes among the published papers. Subdomains for “purpose” and their percentages and references are illustrated in Table 3.
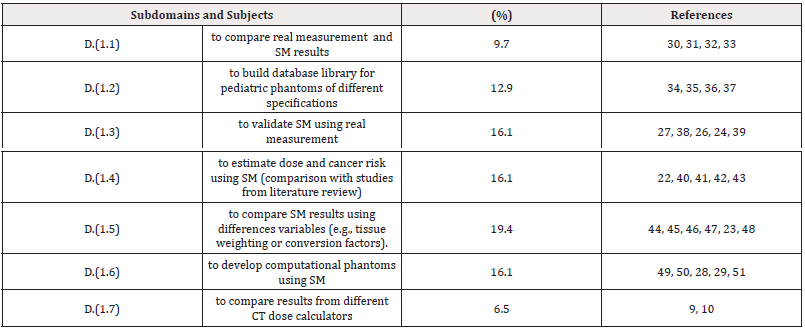
Table 3: Subjects, percentages and references for subdomains: D.(1.1), D.(1.2), D.(1.3), D.(1.4), D.(1.5), D.(1.6), and D.(1.7).
Domain D. (2): (2) is the domain of methodology for the included published papers in this systematic review. D.(2) includes nine subdomains. The subdomains are D.(2.1), D.(2.2), D.(2.3), D.(2.4), D.(2.5), D.(2.6), D.(2.7), D.(2.8) and D.(2.9). Selection of the subdomains subjects was regarding frequent methodologies among the published papers. Subdomains for “methodology” and their percentages and references are illustrated in Table 4.

Table 4: Subjects, percentages and references for subdomains: D. (2.1), D.(2.2), D.(2.3), D.(2.4), D.(2.5), D.(2.6), D.(2.7), D.(2.8) and D.(2.9).
Domain D. (3): (3) is the domain of finding for the included published papers in this systematic review. D.(3) includes five subdomains. The subdomains are D.(3.1), D.(3.2), D.(3.3), D.(3.4) and D.(3.5). Selection of the subdomains subjects was regarding frequent findings among the published papers. Subdomains for “finding” and their percentages and references are illustrated in Table 5.
Discussions
Pediatric patients are more radiosensitive than adults and their life expectancy is longer. Thus, it is essential to assess the radiation dose from different radiological procedures accurately. A wide internet database search was conducted for the period from January 1990 to September 2023 to find published papers in the “contribution of SM to estimation pediatric radiation dose for abdominal and pelvic CT procedures”. In this systematic review, pediatric age range was assumed to be from birth to 16 years old.
It was found that the results with the SM conditions were comparable. For example, the difference in results between Palareti, et al. [25] and Kost, et al. [26] was less than 20%. However, the difference in results between Fujii, et al. [24] and Pan, et al. [28] was significant (approximately 45%). This is because of different specifications of the simulated CT scanners and phantoms. High organ doses reported by Pan, et al. [28] was because of youngest age used phantom and consequently there were small organs sizes and high organ doses. Thus, it is clear that simulated phantom and scanner characteristics strongly affect the SM results.
The subjects for purpose among the included papers were distributed approximately equally. For example, among the included papers, the purposes of subjects of D.(1.3) ” to validate SM using real measurement” [27,38,26]. D.(1.4) ” to estimate dose and cancer risk using SM” [22,40,41], and D.(1.6) “to develop computational phantoms using SM” [49,50,28]. were 16.1% for each one of them. However, D. (1.7), which was “to compare results from different CT dose calculators” [9,10,] had the lowest percentage (7%). Therefore, more studies are required to be conducted, to compare results from different CT dose calculators. This is to find the differences in accuracy of these CT dose calculators and to contribute to their development.
The subjects for methodology among the included papers varied significantly. For example, using “voxelized phantoms” was 41.9% [22,26,44,45] whereas using “stylized phantoms” was 3.2% [33]. In addition, the percentage of using simulation codes (e.g., MCNP and Geant4) was 77.4% [22,23,26,44,45] whereas using CT dose calculators was 22.6% [9,10]. However, development of CT dose calculators started significantly in 2008, whereas using simulation codes started approximately two decades earlier. Nevertheless, regarding the complexity of using simulation codes, significant progress and easiest use of CT dose calculator software, it is expected that using CT dose calculators will be dominant for assessing CT dose in the near future.
The assessed radiation dose types among the included papers were effective and equivalent or absorbed doses. SM estimation of equivalent and absorbed doses dominated with 81%, whereas estimation of effective dose was 19%. However, it is easy to obtain the effective dose, if the equivalent or absorbed dose is known, whereas the opposite cannot be performed. This is because effective dose is a summation of all equivalent doses, as well as equivalent dose is absorbed dose multiplies by tissue weighting factor for each tissue or organ [52]. Thus, it is recommended to obtain simulated organ radiation dose (i.e. equivalent or absorbed), rather than effective radiation dose.
In this systemic review, 74.2% of the SM studies reported that simulated results were strongly related to simulated CT scanner or phantom characteristics [9,10,22,24,26,27]. Also, it was reported that under the same conditions, there were agreement in results using simulated and real measurement methods [24,28,38]. In addition, it was reported that under the same conditions, there were agreement in results using simulation code and CT dose calculator [23,32]. Thus, different simulation codes, CT dose calculators and real measurements are comparable in their results for the same applied conditions. Nevertheless, few studies reported that there was variation in results using different CT dose calculator types [9,10]. Therefore, more studies are required to be conducted to compare results from different CT dose calculators.
A few studies examined the difference in results for different variables that were applied in simulation codes or CT dose calculators, such as, tissue weighting factors, conversion factors (e.g., DLP to effective dose conversion factors). For example, it was reported that the dose to pelvis was found to be 6.5% higher if using ICRP publication 103 recommendations rather than using ICRP publication 60 recommendations, whereas it was lower for all other examinations [44]. Another study found that conversion factors were lower by 33% and 32% than the published data for ICRP publication 60 and ICRP publication 103, respectively [46]. Only one SM study was found in radiation dose estimation of blood circulation and cancer risk estimation [22]. Thus, it is recommended to conduct more SM studies (1) to assess using different conversion and tissue weighting factors and (2) to estimate cancer risk from pediatric abdominal and pelvic CT procedures.
Finally, some studies highlighted their limitations, for example estimation of radiation dose was performed for certain pediatric age or CT scanner type. However, estimation of radiation dose independent on CT scanner type are broadly used in many CT dose calculators [8,53]. Another study highlighted that SM was performed with fixed exposure factor (i.e., mAs) [26,30,34]. To overcome this limitation and to avoid imprecise radiation dose estimation, tube current modulation (TCM) is recommend rather fixed tube current or exposure factors [54]. On the other hand, some studies highlighted their advantages of wide variation in pediatric phantom models, recommendation for development of conversion factors and recommendation for using certain phantom type (e.g., voxelised phantom) [31,46,49].
Conclusions
It can be concluded that SM results with the same conditions are comparable, and the efficiency of different SM were approximately the same (i.e., codes and CT dose calculators). However, it is expected that using CT dose calculators will be dominant method for assessing CT dose in the near future. This is because of the significant progress achieved in these CT dose calculators and the ease of use, compared to simulation codes. Even though Adequate studies were found, conducting studies for specific subjects are recommended.
Recommendations
It is recommended to obtain simulated organ doses rather than simulated effective, as it is easy to drive the effective dose, if the equivalent or absorbed dose is obtained, whereas the opposite cannot be performed. More studies are recommended to investigate the results of SM with applying different variables, such as tissue weighting factors and conversion factors. Additional studies are recommended to compare the results from different CT dose calculators. This is to find the accurate difference in their results and to contribute significantly to the development of SM. Also, it is useful to conduct studies in estimation of radiation dose for blood circulation and cancer risks.
Financial Support and Sponsorship
None.
Conflict of Interests
There is no conflict of interest in this study.
References
- Kleinerman RA (2006) Cancer risks following diagnostic and therapeutic radiation exposure in children. Pediatr Radiol 36(2): 121-125.
- Hall EJ (2009) Radiation biology for pediatric radiologists. Pediatr Radiol 39 Suppl 1: S57-64.
- Aloufi KM, Alhazmi FH, Abdulaal OM, Qurashi AA (2021) Towards the Establishment of Diagnostic Reference Levels in Saudi Arabia: Review and Opinion. Egypt J Radiat Sci Appl 34(1).
- Rixe J, Conradi G, Rolf A, A Schmermund, A Magedanz, et al. (2009) Radiation dose exposure of computed tomography coronary angiography: Comparison of dual-source, 16-slice and 64-slice CT. Heart 95(16): 1337-1342.
- Sobol’ IM (2018) A Primer for the Monte Carlo Method. CRC Press.
- DeMarco JJ, Cagnon CH, Cody DD, M Zankl, E Angel, et al. (2007) Estimating radiation doses from multidetector CT using Monte Carlo simulations: Effects of different size voxelized patient models on magnitudes of organ and effective dose. Phys Med Biol 7;52(9): 2583-2597.
- Lee C, Kim KP, Long D, Ryan Fisher, Chris Tien, et al. (2011) Organ doses for reference adult male and female undergoing computed tomography estimated by Monte Carlo simulations. Med Phys 38(3): 1196-1206.
- Lee C (2021) A Review of Organ Dose Calculation Tools for Patients Undergoing Computed Tomography Scans. J Radiat Prot Res 46(4): 151-159.
- Ding A, Gao Y, Liu H, Bob Liu, X George Xu, et al. (2015) VirtualDose: A software for reporting organ doses from CT for adult and pediatric patients. Phys Med Biol 21;60(14): 5601-5625.
- Abdullah A, Sun Z, Pongnapang N, Ng KH (2012) Comparison of computed tomography dose reporting software. Radiat Prot Dosimetry 151(1): 153-157.
- Rogers DWO (2006) Fifty years of Monte Carlo simulations for medical physics. Phys Med Biol 7 51(13): R287-301.
- Agostinelli S, Allison J, Amako K, H. Araujo, D. Axen, et al. (2003) Geant4-a simulation toolkit. Nucl Instruments Methods Phys Res Sect A Accel Spectrometers, Detect Assoc Equip 506(3): 250-303.
- Team X-5 MC (2008) MCNP--A General Monte Carlo N-Particle Transport Code, Version 5 836.
- Battistoni G, Boehlen T, Pik Wai Chin, Cerutti F, Vasilis Vlachoudis, et al. (2015) Overview of the FLUKA code. Ann Nucl Energy 82: 10-18.
- Bielajew AF, Hirayama H, Nelson WR, Rogers DWO (1994) History, overview and recent improvements of EGS4 National Laboratory for High Energy Physics. History (June).
- Boone JM, McNitt-Gray MF, Hernandez AM (2017) Monte Carlo Basics for Radiation Dose Assessment in Diagnostic Radiology. J Am Coll Radiol 14(6): 793-794.
- Boone JM, Nelson TR, Lindfors KK, Seibert JA (2001) Dedicated Breast CT: Radiation Dose and Image Quality Evaluation. Radiology 221(3): 657-667.
- Ay MR, Sarkar S, Shahriari M, Sardari D, Zaidi H, et al. (2005) Assessment of different computational models for generation of x-ray spectra in diagnostic radiology and mammography. Med Phys 32(6): 1660-1675.
- Tekin HO, Altunsoy EE, Kavaz E, Sayyed MI, Agar O, et al. (2019) Photon and neutron shielding performance of boron phosphate glasses for diagnostic radiology facilities. Results Phys 12: 1457-1464.
- Noujeim M, Prihoda TJ, Langlais R, Nummikoski P (2009) Evaluation of high-resolution cone beam computed tomography in the detection of simulated interradicular bone lesions. Dentomaxillofacial Radiol 38(3): 156-162.
- Deak P, van Straten M, Shrimpton PC, Zankl M, Kalender W (2008) Validation of a Monte Carlo tool for patient-specific dose simulations in multi-slice computed tomography. Eur Radiol 18(4): 759-772.
- Franck C, Vandevoorde C, Goethals I, Klaus Bacher, Peter Smeets, et al. (2016) The role of Size-Specific Dose Estimate (SSDE) in patient-specific organ dose and cancer risk estimation in paediatric chest and abdominopelvic CT examinations. Eur Radiol 26(8): 2646-2655.
- Lee C, Kim KP, Bolch WE, Moroz BE, Folio L (2015) NCICT: a computational solution to estimate organ doses for pediatric and adult patients undergoing CT scans. J Radiol Prot 35(4): 891-909.
- Fujii K, Nomura K, Muramatsu Y, K Takahashi, M Satake, et al. (2015) Evaluation of organ doses in adult and paediatric CT examinations based on Monte Carlo simulations and in-phantom dosimetry. Radiat Prot Dosimetry 165(1-4): 166-171.
- Palareti G, Legnani C, Cosmi B, N Erba, S Testa, et al. (2016) Comparison between different D-Dimer cutoff values to assess the individual risk of recurrent venous thromboembolism: Analysis of results obtained in the DULCIS study. Int J Lab Hematol 38(1): 42-49.
- Kost SD, Fraser ND, Carver DE, Michael G Stabin, Marta Hernanz-Schulman, et al. (2015) Patient-specific dose calculations for pediatric CT of the chest, abdomen and pelvis. Pediatr Radiol 45(12):1771-1780.
- Akhlaghi P, Miri-Hakimabad H, Rafat-Motavalli L (2015) Dose estimations for Iranian 11-year-old pediatric phantoms undergoing computed tomography examinations. J Radiat Res 56(4): 646-655.
- Pan Y, Qiu R, Gao L, Junli Li, Chaoyong Ge, et al. (2014) Development of 1-year-old computational phantom and calculation of organ doses during CT scans using Monte Carlo simulation. Phys Med Biol 21;59(18): 5243-5260.
- Staton RJ, Lee C, Lee C, Matt D Williams, David E Hintenlang, et al. (2006) Organ and effective doses in newborn patients during helical multislice computed tomography examination. Phys Med Biol 21;51(20): 5151-5166.
- Hardy AJ, Bostani M, Kim GHJ, Cagnon CH, Zankl MA, et al. (2021) Evaluating Size-Specific Dose Estimate (SSDE) as an estimate of organ doses from routine CT exams derived from Monte Carlo simulations. Med Phys 48(10):6160-6173.
- Kostou T, Papadimitroulas P, Papaconstadopoulos P, Devic S, Seuntjens J, et al. (2019) Size-specific dose estimations for pediatric chest, abdomen/pelvis and head CT scans with the use of GATE. Phys Medica 65: 181-190.
- Bostani M, McMillan K, Lu P, John J DeMarco, Chris H Cagnon, et al. (2017) Estimating organ doses from tube current modulated CT examinations using a generalized linear model. Med Phys 44(4):1500-1513.
- Stepusin EJ, Long DJ, Ficarrotta KR, Hintenlang DE, Bolch WE (2017) Physical validation of a Monte Carlo-based, phantom-derived approach to computed tomography organ dosimetry under tube current modulation. Med Phys 44(10): 5423-5432.
- Carver DE, Kost SD, Fraser ND, Nicholas D Fraser, Michael G Stabin, et al. (2017) Realistic phantoms to characterize dosimetry in pediatric CT. Pediatr Radiol 47(6): 691-700.
- Lee C, Kim KP, Long DJ, Bolch WE (2012) Organ doses for reference pediatric and adolescent patients undergoing computed tomography estimated by Monte Carlo simulation. Med Phys 39(4): 2129-2146.
- Akhlaghi P, Miri Hakimabad H, Rafat Motavalli L (2015) Evaluation of dose conversion coefficients for an eight-year-old Iranian male phantom undergoing computed tomography. Radiat Environ Biophys 54(4): 465-474.
- Lee C, Lee C, Staton RJ, David E Hintenlang, Wesley E Bolch, et al. (2007) Organ and effective doses in pediatric patients undergoing helical multislice computed tomography examination. Med Phys 34(5): 1858-1873.
- Carver DE, Kost SD, Fernald MJ, D R Pickens, R R Price, et al. (2015) Development and validation of a GEANT4 radiation transport code for CT dosimetry. Health Phys 108(4): 419-428.
- Tzedakis A, Damilakis J, Perisinakis K, Karantanas A, Karabekios S, et al. (2007) Influence of z overscanning on normalized effective doses calculated for pediatric patients undergoing multidetector CT examinations. Med Phys 34(4): 1163-1175.
- Theocharopoulos N, Damilakis J, Perisinakis K, Tzedakis A, Karantanas A, et al. (2006) Estimation of effective doses to adult and pediatric patients from multislice computed tomography: A method based on energy imparted. Med Phys 33(10): 3846-3856.
- Suliman II, Khamis HM, Ombada TH, Alzimami K, Alkhorayef M, et al. (2015) Radiation exposure during paediatric CT in Sudan: CT dose, organ and effective doses. Radiat Prot Dosimetry 167(4): 513-518.
- Khursheed A, Hillier MC, Shrimpton PC, Wall BF (2002) Influence of patient age on normalized effective doses calculated for CT examinations. Br J Radiol 75(898): 819-830.
- Gao Y, Quinn B, Pandit-Taskar N, Jean St Germain, Lawrence T Dauer, et al. (2018) Patient-specific organ and effective dose estimates in pediatric oncology computed tomography. Phys Medica 45: 146-155.
- Caon M (2013) The ratio of ICRP103 to ICRP60 calculated effective doses from CT: Monte Carlo calculations with the ADELAIDE voxel paediatric model and comparisons with published values. Australas Phys Eng Sci Med 36(3): 355-362.
- Tian X, Li X, Segars WP, Frush DP, Paulson EK, et al. (2013) Dose coefficients in pediatric and adult abdominopelvic CT based on 100 patient models. Phys Med Biol 21;58(24): 8755-8768.
- Deak PD, Smal Y, Kalender WA (2010) Multisection CT Protocols: Sex- and Age-specifi c Conversion Dose from Dose-Length Product. Radiology 257(1): 158-166.
- Shrimpton PC, Jansen JTM, Harrison JD (2016) Updated estimates of typical effective doses for common CT examinations in the UK following the 2011 national review. Br J Radiol 89(1057): 20150346.
- Schlattl H, Zankl M, Becker J, Hoeschen C (2012) Dose conversion coefficients for paediatric CT examinations with automatic tube current modulation. Phys Med Biol 21;57(20): 6309-2636.
- Lee C, Williams JL, Lee C, Bolch WE (2005) The UF series of tomographic computational phantoms of pediatric patients. Med Phys 32(12): 3537-3548.
- Xie T, Kuster N, Zaidi H (2017) Computational hybrid anthropometric paediatric phantom library for internal radiation dosimetry. Phys Med Biol 21;62(8): 3263-3283.
- Akhlaghi P, Miri-Hakimabad H, Rafat-Motavalli L 2015) Dose estimation in reference and non-reference pediatric patients undergoing computed tomography examinations: A Monte Carlo study. Radioprotection 50(1): 43-54.
- McCollough CH, Schueler BA (2000) Calculation of effective dose. Med Phys 27(5): 828-837.
- Turner AC, Zankl M, DeMarco JJ, John J DeMarco, Chris H Cagnon, et al. (2010) The feasibility of a scanner-independent technique to estimate organ dose from MDCT scans: Using CTDIvol to account for differences between scanners. Med Phys 37(4): 1816-1825.
- Giansante L, Martins JC, Nersissian DY, Paulo R Costa, Karen C Kiers, et al. (2019) Organ doses evaluation for chest computed tomography procedures with TL dosimeters: Comparison with Monte Carlo simulations. J Appl Clin Med Phys 20(1): 308-320.