Case Report
Creative Commons, CC-BY
Improving Diagnosis in Pediatric Orthopedics Surgery Using 3D Printing
*Corresponding author: Leonardo Frizziero, Department of Industrial Engineering, Alma Mater Studiorum University of Bologna, Italy
Received: August 14, 2019;Published: August 29, 2019
DOI: 10.34297/AJBSR.2019.04.000860
Abstract
In this paper is presented a new methodology for the diagnosis of flat feet in orthopedic application. The resulting new workflow will guarantee higher efficiency reducing costs and time. In particular an economic valuation of both workflows is performed showing the reduction of costs with the methodology presented. Through this elaborate it will be shown that today’s most widely used medical technique for the diagnosis and treatment of congenital flat foot from tarsal synostosis have some weaknesses that could be optimized. To reduce weaknesses, the alternative methodology presented involves a new technology within the traditional method’s workflow. In Orthopedics, as in other fields of medicine, new technologies are introduced aiming to improve the patient healthcare. These technologies allow to perform the same treatments in a simpler and faster way making them much more effective and precise. The technology introduced In Proposed methodology is the 3D Printing.
Keywords: 3D printing; Diagnostics; Orthopedics; Pediatrics; Radiology; Additive manufacturing
Introduction
Surgical planning is evolving step by step with the improvement of the technology available for diagnostic imaging. In The early 1900 ‘ the only diagnostic imaging technique used was the radiology. Nowadays there are modern systems of Acquisition as the Computerized tomography (TC) that provide the surgeon with detailed reconstructions of the patient’s anatomy. The proposed methodology wants to take a further step forward, by transferring the planning from the virtual to the physical world thanks to the printing of three-dimensional anatomic parts. The Workflow To get to the printing of anatomic models start from Tomographic image acquisition through the traditional non-invasive acquisition technique, the TC. Then, a series of software are needed to convert these images in a CAD-readable file format for the 3D printing software [1].
The development of these models can be a challenge because of the complexity and irregularity of the human anatomy and therefore requires the collaboration of competent figures both in the medical and in the Engineering field. There are a variety of solutions available for the conversion. This paper describes the procedure using a combination of 3 of them: InVesalius, MeshLab and Meshmixer. This study involves a sample of 10 patients of 25 that annually undergo resection of the tarsal synostosis from SC Orthopedics and traumatology. The final goal of the paper is to demonstrate the impact of this innovative procedure from the economic point of view [2]. During the pilot study we saw that the proposed model has advantages for patients, doctors and hospitals. For the patient there is a better understanding of the clinical situation, a tailored, safer and less invasive surgical treatment. The doctor benefits from a better perception thanks to the possibility of simulating surgery and predicting the outcome of the intervention. The hospital has reduced risks of complications or infections.
In this paper a SWOT Analysis was performed to analyze the innovative procedure, to discuss its strengths, weaknesses, external threats and possible future developments [3]. It should be noted that 3D printing is used in the medical field with countless purposes including the creation of prostheses and the use of reconstructions for educational purposes. However, in this paper we will only use the 3D printing technique for the three - dimensional reproduction of feet of patients suffering from the disease of the flat foot from tarsal synostosis in order to improve the process of diagnosis and treatment of the specific pathology. The state of the art was carried out based on the well know flat feet pathology [4] and the 3D model steps were tested for a different geometry case to allow a better time/cost analysis.
State of the Art of Diagnosis and Treatment of Flat Foot Pathology
Diagnosis
The flat foot is a common childhood condition which can cause pain and an altered gait. This pathology is determined by the fall of the plantar arch and the valgus pronation of the heel. It tends to lean towards the inside and the rest of the foot tends to point outwards causing the pathology (Figure 1&2) [5,6].
In the first phase of walking, up to 3 years, this condition is completely normal and is part of the physiological growth of the foot. After the age of 3, if the plantar arch isn’t correctly formed, an external action is required such as the use of an arch support. If this does not lead to an improvement of the plantar vault within 8-9 years, corrective surgery is recommended to be performed between 9 and 14 years. The flat foot determines an imbalance of the load at the level of the foot and an imbalance at the level of the posture, therefore can determine the occurrence of painful symptoms. Focusing on subjects of both sexes in infancy-adolescence age (8-16 years) suffering from congenital flat foot from tarsal synostosis, (Figure 3) shows the workflow of the traditional procedure. The main radiological procedure applied are: Radiology (RX) and Computed Tomography (CT). All radiological imaging techniques generally use X-ray beams to represent anatomical structures. The images generated by these methodologies are used to verify the presence of possible pathologies in the anatomical portion subjected to analysis. Of course, X-Ray exposition continues to represent a risk factor for the patient. X- rays produce ionizing radiation, a potentially harmful form of radiant energy. It must be emphasized that the risk of developing cancer following exposure to radiation it is generally contained and depends on at least two factors:
Dose of the radiation: the risk increases as a function of dose and frequency to whom the patient is subjected. Moreover, the risk is greater in young subjects, exactly the ones considered in this paper. Applying the new workflow will rather decrease the number of X-Ray a patient should receive [7]. However, X-ray has some limits concerning the information it provides, in fact these are limited to the bone tissue and lack three-dimensionality. Therefore, the result of first level investigation that outcomes of RX may not be sufficient to understand if the patient is suffering from the pathology and 2D images aren’t clear enough to allow the doctor to decide whether to intervene surgically or not. In the case of tarsal synostosis, the main exam for the diagnosis and the choice of the best treatment, both conservative and surgical, is CT. The patient will then be subjected to second level investigations [8]. Unlike the first-level exam, the CT exam produces 360° images around the body, therefore exposure to X-rays occurs for a longer period. Even the ‘radiation exposure from CT acts differently on children and adults. Children are significantly more sensitive to radiation. In patients subjected to multiple CT before the age of 15, major risks of leukemia, brain tumors and other cancers were found in the decade after the first CT scan, however the overall risk of cancer due to a single CT scan was shown to be low , approximately one case per 10,000 procedures performed in children.
Obtained the tomographic images these are studied in order to carry out a pre-operative planning in which such images are fundamental to define the precise location and orientation of the cut (Figure 3)[4]. Since tomographic images derive from the overlap of 2D images, they can confuse in determining how to proceed with the correct cut of the synostosis. They can also make it difficult to understand how thick the synostosis to be resected is to avoid injury to nearby bones and joints behind the lesion. Considering what was said about exposure to X-rays, the benefit is obvious to find a method that optimizes these first phases both in terms of minimizing the number of radiological examinations and making the tools used in the preoperative planning more efficient. Furthermore, from an economic standpoint, for every RX less the saving is around 22 € while this arise to 87€ in case of CT. (Table 1) shows the overview of the costs and risk of the various procedures that compose this first phase of diagnostic.
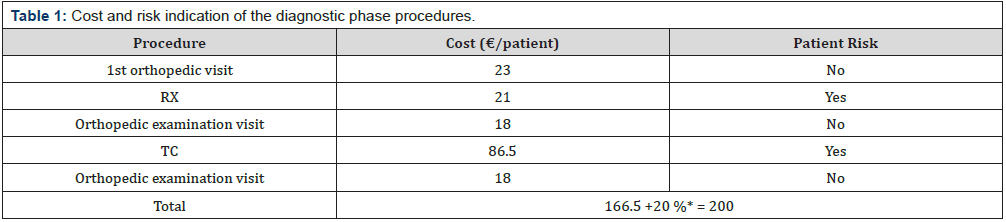
Table 1: Cost and risk indication of the diagnostic phase procedures.
Note: * + 20% indicates the addition, the specific costs of the procedure, due to administrative and general costs.
Surgical operation
Once the phase of diagnosis is over, we proceed to the surgical operation. During preoperative planning, the most widely used methodology to date is CT, but this is not enough to guide the medical team during surgery. For this reason, the fluoroscope is needed in the operating room. Once again, we use X-rays, this time to try to fill the doubts and uncertainties left by the study of only tomographic images. It would therefore be appropriate for the safety of both the patient and the surgeon, use a technology that monsters from the outset in a manner clear to the anatomy of the malformed foot thus allowing the specialist to intervene with greater safety, also reducing the period of use of the fluoroscope. After resection of the synostosis, the operation continues as in (Figures 4&5) respectively show the step of resection of the synostosis and that of interposition of the material. In some cases, the imperfect outcome of an operation requires a possible surgical revision, the patient will therefore have to undergo an operation very similar to the main one, or to a further CT scan. The costs have also been summarized for this phase (Table 2) which involve the various procedures performed. As can be seen in the, the duration of an intervention of this type is approximately 60 minutes and its cost around €4050.

Table 2: Cost and risk indication of the intervention phase procedures.
Note 1: The cost of the first hospitalization also includes the cost of the plaster room.
Note 2: + 20% indicates the addition, to the specific costs of the procedure, due to administrative and general costs.
Post-Operative Stage
For a correct post-operative course, a sequence of 5 orthopedic check-ups are required. These are carried out with a time interval of about 3 months from each other. If after the first 4 visits no problems occurs, during the last visit the removal of the screws will take place, a procedure that requires a small intervention in Day Surgery. The cost of the post-operative stage is listed in (Table 3). (Table 4) summarize the traditional methodology emphasizing the main issues that have been identified during the analysis of the traditional technique.
The New Workflow
Model Creation
As mentioned in the previous paragraph, the images extrapolated from the CT are not always sufficiently clear to allow the surgeon to elaborate in detail the procedure to be performed before the operation. Accordingly, to the new workflow, a 3d model from CT scan should be produced. This model may be used to during the study phases of the operation and/or during the surgical operation itself. The primary goal that to achieve thanks to the three-dimensional anatomical reproduction is the reduction of the duration of the surgical procedure, with consequent reduction of anesthesiologic times and surgical wounds. Moreover, the reduction of fluoroscope in the operating room should occurs. The process can be subdivided in six consecutive steps (Figure 6).
From CT to 3D
The methodology proposed in this paper uses tomographic images as a starting point for generate a three-dimensional model. Thanks to this technique, the density of tissues crossed by X-rays is converted into different gray levels. The program in Vesalius was chosen for this purpose as in it is a free medical software. It is able to generate virtual reconstructions of structures of human body based on CT scans. The first operation to be performed in in Vesalius is importing a DICOM format file (Figure 7)[8].
Following the import, the software allows to visualize the biological structure of interest in the three main axes of the human body (Figure 8)[10]. The next step is to segment the image using a colored mask. The mask is nothing but gray threshold level comparable to a certain tissue density. By setting the density range, the software highlights a specific region and creates its three-dimensional model, eliminating the rest. There is the possibility of pre-defined range (for example: compact bone, spongy bone, skin, muscular tissue etc.) or it can be changed manually. After the correct identification of the mask, the 3D surface is generated using the proper command (Figure 9). In the analyzed case it was preferred to use the “ Compact bone (adult) “ because the range was more limited avoiding other possible tissue inclusion. When the mask covers the right areas, the creation of the three-dimensional surface is performed. This is displayed in the lower right window (Figure 10). Once the 3D surface is created and exported in STL format for further modifications. Generally, the mesh created by In Vesalius is coarse and not homogeneous, so it needs an optimization process.
The Optimization Process
For shape optimization was used MeshLab. In (Figure 11) it is possible to observe only the representation of the tibia. The inaccuracies and irregularities mentioned above are clear here, especially in the upper part of the bone, near the knee. Furthermore, in the lower part of the image there is the indication relative to the number of faces that make the 3D surface (589951). To reduce the number of triangles that compose the mesh, it is important to eliminate vertices and faces included in the inner cavity of the model. These are useless for the purpose 3D printing. Their elimination simplifies the geometry making the 3D-printing phase faster and more effective. The Ambient Occlusion philter is applied which highlights the visible part. This function simulates a global diffuse illumination and colors the faces of the mesh according to a gray scale based on how much the single polygon is exposed to illumination. Once the philter is applied, we proceed selecting the bone inner material (highlighted in red in (Figure 12)) in order to simplify the model.
(Figure 13) shows the empty bone interior and the number of faces from which the optimized model is composed: 268349. Moreover, the elimination of small and isolated surfaces needs to be performed. Small Components function is used. This philter allows to choose the Ratio below which surfaces should be delated (Figure 14).At the end of the optimization process, mesh irregularities such as:
a. Duplicate triangles
b. Non- manifold edges
c. Non- manifold vertices
d. Orientation problems: the triangles are not oriented in the same direction
e. Disconnected zones: the mesh is composed of several disconnected zones are removed and the resulting clean surface will be used in the 3D printing process (Figure 14&15).
Because of the dimension of this particular bone (X = 91.34 mm, Y = 372 mm and Z = 77.58 mm) (Figure 16), MeshMixer is used to separate the geometry in two parts and generate a connection pin (Figure 17). The 3D printer used for this application is an FDM (Fused Deposition Modeling) technology, EZT3D Delta (Figure 18). In (Table 5) a material cost analysis was carried out based on the current price on market of the specific material per kg. Moreover, the autoclavability was considered despite the cost because of the particular medical application. Finally, the methodology studied, allowed to obtain a three-dimensional bone model in PLA that perfectly fits the anatomical structure of the CT images (Figure 19).
Cost Analysis
The realization of the three-dimensional model involves a series of costs:
1. Purchase of the 3D printer
2. Labor cost
3. Material cost
4. Software
The costs relative to this specific printed model are summarized in (Table 5). The cost of the printer is about 220 € and its useful life is approximately of 2000 working hours. The labor time required for the conversion and the cleaning of the model is 4 hours average and the estimated cost of the specialized operator is 20 €/h. For the printing of the model it is necessary an average of 0,250 kg of material. The actual printing time to get the 3D model is 22 hours. The software used in the proposed procedure are all open source and they don’t imply added costs.
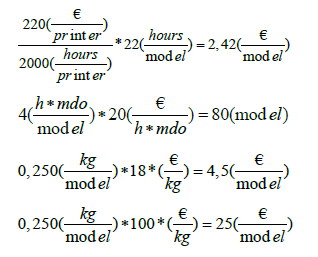
The Innovative Workflow
For the survey we follow the data obtained by analyzing the pilot study [“3D-printing models in the pre-operative planning of the pediatric patient suffering from congenital malformations”][11]. Thanks to 3D printing, the surgeon can better understand the anatomical condition of the patient. The possibility of developing more detailed surgical plans allows the doctor to achieve better results, safety and speed during the operation. The possibilities to refer to the prototype both before and during the intervention should allow a minor use of the fluoroscope. About the post-operative stage, controls are still performed because they are necessary to ensure that the patient is responding well to the operation. The new workflow is illustrated in (Figure 20).
It is observed that, using the prototype during surgical planning, the duration of the intervention is reduced. Decreasing the intervention times allows a lower use of anesthetic dose for the patient lower risk of infection.
The use of 3D models during the pre-operative phase let surgeon proceed with more confidence during surgery due to increased knowledge of the anatomy of that specific case. One of the main advantages of this new method is the radiation time reduction [12]. With the new procedure the first RX scan is no more necessary, and the use of fluoroscopy should be reduced. In addition, the patient could learn the procedure to which he will be subjected interactively through a simulation on the prototype. All this has a positive impact on the hospital as it guarantees an improvement in the service for both patients and hospital staff. There is also a decrease in direct costs. The advantages obtained are summarized in (Table 7).
(Table 8) shows that problems of the traditional method are now solved with the introduction of three-dimensional anatomical model:
However, even the innovative procedure is not without limitations. The most obvious is the difficulty of organizing all the steps in the workflow. The conversion of the tomographic images in a STL file should be performed as soon as possible and sent directly to the 3D printer. Another weakness is the correct creation of three-dimensional surface. The modification of the mesh is carried out manually and the operator should have both engineering than medical skills. If this condition is not achieved, higher risks of model inaccuracy can occur, and the model could be useless. Particularly, mesh cleaning is the riskiest step because it needs high awareness of bone anatomy and patient pathology to guarantee a correct 3D surface.
Innovative Procedure Profits
Using a three-dimensional reproduction with the new workflow allows to save money in a cost analysis. In the diagnosis phase the number of orthopedic visits fell from 3 to 2 for each patient decrease by 33.4% or saving 18 €/patient. Moreover, the 3D model allows to bypass the first radiological step so the number of radiological visits per patient is halved avoiding the expense of 21 €/ patient. The traditional surgical intervention was about 60 minutes average. Using the prototype, the time frame should be reduced by 15 minutes or 25% less This reduction in use of the operating room costs 206.75 €/ patient less. Finally, studying the case on the 3D prototype decrease the recurrence rate of 15% compared to the traditional case (only CT used). Moreover, with the innovative methodology, one less case of relapse in a year occurs (2 cases compared to 3 in the traditional procedure). In the worst-case scenario, if all 2 recidivist patients need re-intervention, the total saving is about 5094.32€ (new TC: 86.5 €; inspection visit: 18 €; the surgery: 3380 €; post-operative phase: 1627.32 €). (Table 9) summarizes the improvement rates of the new workflow in terms of money saving per year. Looking at the (Figure 21), the saving in the diagnosis phase is 1295,4 €/year, in the surgery phase it is 10258.5 €/year while in the post-operative phase a is 1952.8 €/year for a total saving of € 13,526.7 €/year. It results in a total reduction in spending of 7/8 % per year.
It is also necessary to consider the expense due to the cost of creating a sterilizable prototype, i.e 107.42 €/model since the only suitable from the list before mentioned is HT-PLA. Considering that the number of patient’s 3D models in one year is average 27 (25 new cases and 2 repeat offenders), the total cost is approximately 2900 €/year. Finally, the cost of the innovative method is therefore around 10607 €/year, which allows an annual saving of 6.1 %. The result obtained is based on the worst hypothesis for recurrence cases where re-surgery is considered necessary. It was considered in also the case of zero recidivism for both procedures. Taking account that the production cost of 25 prototypes is 2686 €, the total cost of the proposed procedure is 102943 €/year. The advantages brought by the use of the prototype make the innovative procedure more tempting because of the lower costs also in this case.
S.W.O.T. Analysis
Strength
Usually when we talk about innovative technologies, we expect to face high costs for realization and/or use. In the case analyzed in the paper, however, the advantages that the introduction of 3D printing brings to the procedure not only allow to cover the production costs, but also to save money compared to the expense foreseen by the traditional procedure. This is possible not only thanks to the reduction of the times of some activities such as the surgery, but also because the cost of model realization is cheap. The improved quality of the procedure has a strong impact on both the patient and the doctor. The first sees the reduces the number of visits, he achieves greater awareness of his surgery and reduces the exposure to X-rays. The second testing the procedure on the prototype, can well-define the surgical plan more precisely improving the surgical outcome.
Weakness
The coordination between the doctor and the mechanical engineer is a weakness point. The right collaboration is needed for the success of the prototype in order to avoid inconsistencies with the actual anatomy of the bone reproduced. The optimal solution would be to entrust the realization of the model to professional operator expert both in the medical and engineering fields. If the two figures are different, physical proximity could be sufficient to simplify communication solving uncertainty during the 3D surface cleaning phase. Moreover, the introduction of the 3D model within the medical workflow requires a higher awareness of 3D printers and printing materials in order to choose the most suitable combination of them for the specific purpose.
Threat
Surgeons, radiologists and engineers must develop new skills and assume new roles. The risk is that without a specific training of the personnel on used software and hardware could cause a wrong implementation of the new methodology leading to higher costs and times.
Opportunity
One opportunity is the internalization of the prototype realization procedure. Coordination is one of the fundamental factors for the success of the procedure, merging this step in one would improve the workflow.
The 3D printing process could be helpful in realization of patient- specific instrument (PSI) involves the use of CT associated to methods of “rapid prototyping”. A specific cutting guide could be a possible implementation of PSI. Custom tools can increase both the operating accuracy and reduce operating times (Figure 17).
Conclusion
From the traditional methodology study some weakness was pointed out. First of all, the inaccuracy of the techniques used for diagnosis. From this problem, the desire to provide surgeons a tool that allows to better understand the difficulties related to the anatomy of each individual case. The proposed tool is a three-dimensional model that can be used in the planning phase. The use of various software explained before, has proved to be able to achieve a reproduction of a bone in HT-PLA that perfectly reflects the anatomical structure of TC images. The economic impact due to the introduction of this technology into the traditional workflow was studied. The production costs currently required for an autoclavable model are around 110 €/model.
On the other hand, from the comparison between the procedure currently in use and the proposed one, a considerable advantage was found both for the patient and for the doctor. The patient would have a better understanding of his clinical situation and less invasive surgical treatment. The doctor benefits from a better visualization of the problem thanks to the planning of surgery during the pre-operative stage. The hospital would bear lower direct costs and reduced risks of complications or infections. The direct costs are indeed reduced thanks to the considerable advantages brought using the 3D model, first of all the reduction of the intervention time and the recurrence rates.
Considering both the economic savings made and the additional costs necessary for the 3D model, it was concluded that the innovative method is convenient both from a practical and an economic point of view. However, it is important to keep in mind that the research has focused on a very limited number of patients.
Possible future developments have been identified to extend the use of 3D printing in orthopedics in order to improve the operative planning of other pathologies as well. An example is the realization of a 3D printing laboratory inside the hospital, managed by subjects specialized in 3D model for medical purposes [13].
References
- Maredi E, Stilli S (2017) Protocollo.
- Auricchio F, Marconi S (2016) 3D printing: clinical applications in orthopaedics and traumatology. EFORT Open Rev 1(5): 121-127.
- Docquier P L, Paul L, TranDuy V (2016) Surgical navigation in paediatric orthopaedics. EFORT Open Rev 1(5): 152-159.
- Cameron M (2019) Process to Convert DICOM Data to 3D Printable STL Files.
- Ippolito G, Benedetti M Valentini, Caterini R, Farsetti P (2009) Il piede piatto congenito da sinostosi tarsali, controllo a lunga distanza di 10 casi trattati chirurgicamente. GIOT 35: 204-208.
- Pellegrino A (2011) Chirurgia del piede e della caviglia Testo informativo per i pazienti Pp. 1-8.
- Gindro R (2016) TAC, mezzo di contrasto, rischi e altre domande frequenti.
- James B Carr II, Scott Yang, Leigh Ann Lather (2016) Pediatric Pes Planus: A State-of-the-Art Review. Pediatrics 137(3): e20151230.
- Ghidotti C (2018) Cosa sono le stampanti 3D?
- Meomartino L (2018) Tomografia Computerizzata: principi fisici e formazione delle immagini.
- Osti F, Santi G, Neri M, Liverani A, Frizziero L, et al. (2019) CT Conversion Workflow for Intraoperative Usage of Bony Models: From DICOM Data to 3D Printed Models 9(4): 708-720.
- Rizzo ML (2015) La stampa 3D di lesioni corporee: la nuova frontiera del supporto al consenso informato.
- De Marchi L, Ceruti A, Marzani A, Liverani A (2013) Augmented reality to support on-field post-impact maintenance operations on thin structures. Journal of Sensors Pp. 10.